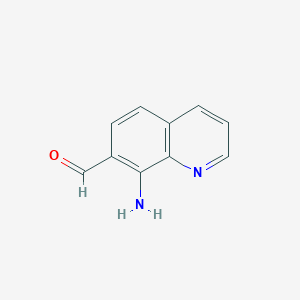
8-Aminoquinoline-7-carbaldehyde
Overview
Description
8-Aminoquinoline-7-carbaldehyde is an organic compound with the molecular formula C10H8N2O It is a derivative of quinoline, featuring an amino group at the 8th position and an aldehyde group at the 7th position
Preparation Methods
Synthetic Routes and Reaction Conditions: The synthesis of 8-Aminoquinoline-7-carbaldehyde typically involves the nitration of quinoline to produce a mixture of 5- and 8-nitroquinoline derivatives. These derivatives are then separated by distillation and sublimation. The 8-nitroquinoline is subsequently reduced using tin powder in the presence of hydrochloric acid to yield 8-aminoquinoline. Finally, the aldehyde group is introduced at the 7th position through formylation reactions .
Industrial Production Methods: Industrial production methods for this compound often involve similar synthetic routes but are optimized for large-scale production. This includes the use of continuous flow reactors and automated separation techniques to enhance yield and purity.
Chemical Reactions Analysis
Types of Reactions: 8-Aminoquinoline-7-carbaldehyde undergoes various chemical reactions, including:
Oxidation: The aldehyde group can be oxidized to form carboxylic acids.
Reduction: The aldehyde group can be reduced to form primary alcohols.
Substitution: The amino group can participate in nucleophilic substitution reactions, forming amides and other derivatives.
Common Reagents and Conditions:
Oxidation: Common oxidizing agents include potassium permanganate (KMnO4) and chromium trioxide (CrO3).
Reduction: Reducing agents such as sodium borohydride (NaBH4) and lithium aluminum hydride (LiAlH4) are frequently used.
Substitution: Reagents like acyl chlorides and anhydrides are used for amide formation.
Major Products:
Oxidation: 8-Aminoquinoline-7-carboxylic acid.
Reduction: 8-Aminoquinoline-7-methanol.
Substitution: Various amides and substituted quinoline derivatives.
Scientific Research Applications
8-Aminoquinoline-7-carbaldehyde has a wide range of applications in scientific research:
Industry: It is used in the synthesis of dyes, pigments, and other industrial chemicals.
Mechanism of Action
The mechanism of action of 8-Aminoquinoline-7-carbaldehyde and its derivatives often involves interaction with biological macromolecules. For instance, in antimalarial applications, the compound targets the liver stages of the malaria parasite, disrupting its lifecycle and preventing relapse . The amino group can form hydrogen bonds with biological targets, while the aldehyde group can participate in covalent bonding, enhancing the compound’s efficacy.
Comparison with Similar Compounds
8-Hydroxyquinoline: Similar structure but with a hydroxyl group instead of an amino group.
Primaquine: An 8-aminoquinoline derivative used as an antimalarial drug.
Tafenoquine: Another antimalarial drug with a longer half-life compared to primaquine.
Uniqueness: 8-Aminoquinoline-7-carbaldehyde is unique due to the presence of both an amino and an aldehyde group, which allows it to participate in a broader range of chemical reactions compared to its analogs. This dual functionality makes it a versatile compound in synthetic chemistry and various applications.
Properties
IUPAC Name |
8-aminoquinoline-7-carbaldehyde | |
---|---|---|
Source | PubChem | |
URL | https://pubchem.ncbi.nlm.nih.gov | |
Description | Data deposited in or computed by PubChem | |
InChI |
InChI=1S/C10H8N2O/c11-9-8(6-13)4-3-7-2-1-5-12-10(7)9/h1-6H,11H2 | |
Source | PubChem | |
URL | https://pubchem.ncbi.nlm.nih.gov | |
Description | Data deposited in or computed by PubChem | |
InChI Key |
ZAMLCNSCTZZXCU-UHFFFAOYSA-N | |
Source | PubChem | |
URL | https://pubchem.ncbi.nlm.nih.gov | |
Description | Data deposited in or computed by PubChem | |
Canonical SMILES |
C1=CC2=C(C(=C(C=C2)C=O)N)N=C1 | |
Source | PubChem | |
URL | https://pubchem.ncbi.nlm.nih.gov | |
Description | Data deposited in or computed by PubChem | |
Molecular Formula |
C10H8N2O | |
Source | PubChem | |
URL | https://pubchem.ncbi.nlm.nih.gov | |
Description | Data deposited in or computed by PubChem | |
DSSTOX Substance ID |
DTXSID00453375 | |
Record name | 8-aminoquinoline-7-carbaldehyde | |
Source | EPA DSSTox | |
URL | https://comptox.epa.gov/dashboard/DTXSID00453375 | |
Description | DSSTox provides a high quality public chemistry resource for supporting improved predictive toxicology. | |
Molecular Weight |
172.18 g/mol | |
Source | PubChem | |
URL | https://pubchem.ncbi.nlm.nih.gov | |
Description | Data deposited in or computed by PubChem | |
CAS No. |
158753-17-4 | |
Record name | 8-aminoquinoline-7-carbaldehyde | |
Source | EPA DSSTox | |
URL | https://comptox.epa.gov/dashboard/DTXSID00453375 | |
Description | DSSTox provides a high quality public chemistry resource for supporting improved predictive toxicology. | |
Record name | 8-Aminoquinoline-7-carboxaldehyde | |
Source | European Chemicals Agency (ECHA) | |
URL | https://echa.europa.eu/information-on-chemicals | |
Description | The European Chemicals Agency (ECHA) is an agency of the European Union which is the driving force among regulatory authorities in implementing the EU's groundbreaking chemicals legislation for the benefit of human health and the environment as well as for innovation and competitiveness. | |
Explanation | Use of the information, documents and data from the ECHA website is subject to the terms and conditions of this Legal Notice, and subject to other binding limitations provided for under applicable law, the information, documents and data made available on the ECHA website may be reproduced, distributed and/or used, totally or in part, for non-commercial purposes provided that ECHA is acknowledged as the source: "Source: European Chemicals Agency, http://echa.europa.eu/". Such acknowledgement must be included in each copy of the material. ECHA permits and encourages organisations and individuals to create links to the ECHA website under the following cumulative conditions: Links can only be made to webpages that provide a link to the Legal Notice page. | |
Synthesis routes and methods
Procedure details
Retrosynthesis Analysis
AI-Powered Synthesis Planning: Our tool employs the Template_relevance Pistachio, Template_relevance Bkms_metabolic, Template_relevance Pistachio_ringbreaker, Template_relevance Reaxys, Template_relevance Reaxys_biocatalysis model, leveraging a vast database of chemical reactions to predict feasible synthetic routes.
One-Step Synthesis Focus: Specifically designed for one-step synthesis, it provides concise and direct routes for your target compounds, streamlining the synthesis process.
Accurate Predictions: Utilizing the extensive PISTACHIO, BKMS_METABOLIC, PISTACHIO_RINGBREAKER, REAXYS, REAXYS_BIOCATALYSIS database, our tool offers high-accuracy predictions, reflecting the latest in chemical research and data.
Strategy Settings
Precursor scoring | Relevance Heuristic |
---|---|
Min. plausibility | 0.01 |
Model | Template_relevance |
Template Set | Pistachio/Bkms_metabolic/Pistachio_ringbreaker/Reaxys/Reaxys_biocatalysis |
Top-N result to add to graph | 6 |
Feasible Synthetic Routes
Q1: How does 8-aminoquinoline-7-carbaldehyde contribute to the synthesis of chiral compounds?
A: this compound serves as a key starting material in the synthesis of chiral ligands. For instance, it reacts with enantiomerically pure nopinone derivatives via Friedländer condensation to yield chiral (2,3-b)-pineno-1,10-phenanthrolines. [] These chiral ligands, upon coordination with Copper(I), form non-interconvertible chiral complexes exhibiting distinct optical properties. [] This demonstrates the utility of this compound in constructing chiral molecules with potential applications in asymmetric catalysis and chiroptical materials.
Q2: What are the common synthetic applications of this compound in material science?
A: this compound acts as a versatile building block for synthesizing diverse spirobifluorene-derived N-heterocycles. [] These compounds, featuring extended π-conjugated systems, exhibit interesting photophysical properties, including strong photoluminescence in the 390–430 nm region. [] Such materials hold promise for applications in organic light-emitting diodes (OLEDs), sensors, and other optoelectronic devices.
Q3: Can you describe the use of this compound in synthesizing organometallic compounds?
A: this compound plays a crucial role in preparing heteroaryl-substituted ferrocenes via the Friedländer reaction. [] Reacting it with diacetylferrocene yields a mixture of mono- and 1,1'-bis(heteroaryl)-substituted ferrocenes, highlighting its versatility in introducing heteroaryl substituents onto the ferrocene core. [] This approach enables the development of novel organometallic compounds with tailored electronic and optical properties for potential applications in catalysis, materials science, and medicinal chemistry.
Disclaimer and Information on In-Vitro Research Products
Please be aware that all articles and product information presented on BenchChem are intended solely for informational purposes. The products available for purchase on BenchChem are specifically designed for in-vitro studies, which are conducted outside of living organisms. In-vitro studies, derived from the Latin term "in glass," involve experiments performed in controlled laboratory settings using cells or tissues. It is important to note that these products are not categorized as medicines or drugs, and they have not received approval from the FDA for the prevention, treatment, or cure of any medical condition, ailment, or disease. We must emphasize that any form of bodily introduction of these products into humans or animals is strictly prohibited by law. It is essential to adhere to these guidelines to ensure compliance with legal and ethical standards in research and experimentation.