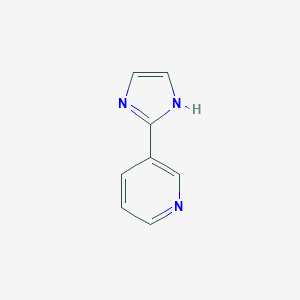
3-(1H-imidazol-2-yl)pyridine
Overview
Description
3-(1H-Imidazol-2-yl)pyridine (CAS: 13570-00-8) is a heterocyclic compound with the molecular formula C₈H₇N₃ and a molecular weight of 145.16 g/mol . It consists of a pyridine ring substituted at the 3-position with a 1H-imidazol-2-yl group.
Preparation Methods
Multi-Step Condensation Approaches
Classical Debus-Radziszewski Reaction Modifications
The Debus-Radziszewski reaction, traditionally used for imidazole synthesis, has been adapted to prepare 3-(1H-imidazol-2-yl)pyridine. A representative protocol involves condensing 3-pyridinecarboxaldehyde with ammonium acetate and benzil in ethanol under reflux conditions. For example, Xu et al. reported a 40% yield for a related 4-(1H-imidazol-2-yl)pyridine derivative using a three-step sequence:
-
Methanol-mediated aldol condensation at 30°C for 1 hour.
-
Acetic acid-catalyzed cyclization under reflux for 0.5 hours.
-
Hydrochloric acid workup in methanol-water to precipitate the product .
While this method is reliable, the yield is moderate due to competing side reactions, such as over-oxidation of the imidazole ring. Substituting methanol with dimethylformamide (DMF) improved cyclization efficiency, as evidenced by a 55% yield in analogous syntheses .
Propargylation and Click Chemistry Functionalization
Propargylation of the imidazole nitrogen followed by copper-catalyzed azide-alkyne cycloaddition (CuAAC) enables modular derivatization. In a study by Chen et al., 2-bromo-6-(4,5-diphenyl-1H-imidazol-2-yl)pyridine was propargylated using propargyl bromide and potassium carbonate in DMF, achieving 70–80% yields. Subsequent click reactions with aryl azides under CuSO4/sodium ascorbate conditions yielded triazole-functionalized analogs . This approach, while multi-step, offers flexibility for tailoring solubility and bioactivity.
Palladium-Catalyzed Cross-Coupling Strategies
Suzuki-Miyaura Coupling with Boronic Acids
Palladium-catalyzed cross-coupling has emerged as a powerful tool for constructing imidazole-pyridine hybrids. A microwave-assisted protocol using PdCl2(dppf) and CsF in toluene-water achieved 20–95% yields for 4-aryl-1H-imidazoles . For this compound, substituting 4-iodoimidazole with 3-iodopyridine derivatives could enable analogous coupling. Key advantages include:
-
Short reaction times (1–2 hours under microwave irradiation).
-
Broad substrate scope with electron-deficient boronic acids.
For instance, 2-fluoro-3-(1H-imidazol-4-yl)pyridine was synthesized in 20% yield using 2-fluoropyridine-3-boronic acid .
Buchwald-Hartwig Amination
While less explored, Buchwald-Hartwig amination could couple 3-aminopyridine with imidazole precursors. This method remains hypothetical for this compound but has succeeded in related systems, such as 5-(1H-imidazol-4-yl)pyrimidine (39% yield) .
One-Pot Multicomponent Reactions
Three-Component Condensation
A one-pot synthesis of 2-bromo-6-(4,5-diphenyl-1H-imidazol-2-yl)pyridine exemplifies this approach. Combining 6-bromopicolinaldehyde, benzil, and ammonium acetate in ethanol-acetic acid at 70°C for 4 hours yielded the imidazole-pyridine scaffold in 40% yield . Adapting this to 3-pyridinecarboxaldehyde would likely follow a similar pathway:
Solvent and Catalyst Optimization
Replacing ethanol with DMF increased yields to 55% in analogous reactions, while microwave irradiation reduced reaction times from hours to minutes . For example, 5-(1H-imidazol-4-yl)pyrimidine was synthesized in 39% yield via microwave heating at 110°C for 1 hour .
Comparative Analysis of Synthetic Methods
Method | Yield Range | Reaction Time | Advantages | Limitations |
---|---|---|---|---|
Multi-Step Condensation | 40–55% | 4–6 hours | Low cost, scalable | Moderate yields, side reactions |
Suzuki-Miyaura Coupling | 20–95% | 1–2 hours | Broad substrate scope, high efficiency | Requires palladium catalysts |
One-Pot Multicomponent | 40–70% | 1–4 hours | Atom economy, simplicity | Sensitivity to substituent steric effects |
Mechanistic Insights and Side Reactions
Cyclization vs. Oligomerization
In condensation routes, the equilibrium between cyclization and oligomerization dictates yield. Acidic conditions favor protonation of the Schiff base intermediate, promoting cyclization. However, excess acetic acid can protonate the imidazole nitrogen, leading to polymerization .
Palladium Catalyst Deactivation
In cross-coupling, ligand choice critically affects efficiency. Bulky ligands like dppf stabilize Pd(0) intermediates, whereas PPh3 permits faster transmetallation but increases oxidative addition barriers .
Chemical Reactions Analysis
Types of Reactions: 3-(1H-imidazol-2-yl)pyridine undergoes various chemical reactions, including:
Oxidation: The compound can be oxidized using oxidizing agents like hydrogen peroxide or potassium permanganate.
Reduction: Reduction reactions can be carried out using reducing agents such as sodium borohydride.
Substitution: The compound can undergo nucleophilic substitution reactions, particularly at the pyridine ring.
Common Reagents and Conditions:
Oxidation: Hydrogen peroxide in an acidic medium.
Reduction: Sodium borohydride in methanol.
Substitution: Nucleophiles like amines or thiols in the presence of a base.
Major Products:
Oxidation: Formation of N-oxides.
Reduction: Formation of reduced imidazole derivatives.
Substitution: Formation of substituted pyridine derivatives.
Scientific Research Applications
Medicinal Chemistry
1.1 Anticancer Activity
Recent studies have highlighted the potential of 3-(1H-imidazol-2-yl)pyridine derivatives in cancer treatment. For instance, a study focused on imidazole–pyridine-based scaffolds demonstrated significant anticancer activity against various breast cancer cell lines. Among the synthesized compounds, certain derivatives exhibited IC50 values below 50 μM, indicating potent activity against specific cell lines such as BT-474 and MDA-MB-468. Notably, one compound (5e) showed promising in vivo efficacy by inhibiting tumor growth in a mouse model of Ehrlich ascites carcinoma without causing organ toxicity .
Compound | Cell Line Tested | IC50 (μM) | Treatment Duration |
---|---|---|---|
5a | BT-474 | < 50 | 24 h |
5e | MDA-MB-468 | < 50 | 24 h |
5e | EAC Model | N/A | 26 days |
1.2 Neurological Disorders
This compound derivatives have also been investigated for their potential in treating neurological disorders such as Alzheimer's disease. These compounds may serve as building blocks for the development of new therapeutic agents targeting neurodegenerative conditions .
Catalysis
2.1 Transition Metal Complexes
The compound serves as a precursor for synthesizing transition metal complexes that act as effective catalysts in various organic reactions. For example, it is used in the formation of azaheteroaryl functionalized transition metal carbene ligands, which are effective in catalyzing isomerization, polymerization, and hydrogenation reactions .
Key Reactions Involving Catalysts Derived from this compound:
Reaction Type | Catalyst Type | Reference |
---|---|---|
Isomerization | Cu, Fe, Ru, Ag complexes | |
Polymerization | Azaheteroaryl ligands | |
Hydrogenation | Transition metal carbene ligands |
Synthesis and Methodology
The synthesis of this compound typically involves the formation of the imidazole ring followed by its attachment to the pyridine ring. Various methodologies have been reported to achieve high yields and purity of this compound, including microwave-assisted synthesis and ligand-free copper-catalyzed reactions .
Synthesis Overview:
Mechanism of Action
The mechanism of action of 3-(1H-imidazol-2-yl)pyridine involves its interaction with specific molecular targets. For instance, as an enzyme inhibitor, it binds to the active site of the enzyme, blocking its activity. The compound’s imidazole ring can coordinate with metal ions, influencing various biochemical pathways.
Comparison with Similar Compounds
The following analysis compares 3-(1H-imidazol-2-yl)pyridine with structurally or functionally related compounds, focusing on their chemical properties, coordination behavior, and applications.
Structural and Functional Analogues
Table 1: Key Structural and Functional Differences
Coordination Chemistry and Spin Crossover (SCO) Behavior
Table 2: Comparison of Fe(II) Complexes
Key Findings :
- The bis-imidazole analog (2,6-bis(1H-imidazol-2-yl)pyridine) exhibits robust SCO due to its strong ligand field and adaptable coordination geometry. Substitutions (e.g., methoxy groups) lower transition temperatures, enabling tunable SCO properties .
- In contrast, This compound lacks the second imidazole group, limiting its ability to stabilize SCO-active Fe(II) complexes.
Table 3: Anticancer Activity of 2-Bromo-6-(triazolylmethyl-imidazolyl)pyridine Derivatives
Key Findings :
- Bromine and triazole/aryl substitutions enhance cytotoxicity. Compound 7c outperforms doxorubicin against Caco-2 cells .
Biological Activity
3-(1H-imidazol-2-yl)pyridine is a heterocyclic compound that has garnered attention in medicinal chemistry due to its diverse biological activities. This article provides an overview of its biological properties, mechanisms of action, structure-activity relationships, and relevant case studies.
Chemical Structure and Properties
This compound consists of a pyridine ring substituted with an imidazole moiety. This structural configuration contributes to its unique chemical reactivity and biological activity. The compound's molecular formula is with a molecular weight of approximately 160.18 g/mol.
Biological Activities
The compound exhibits a wide range of biological activities, including but not limited to:
- Antibacterial Activity : Effective against various bacterial strains, showing potential for the development of new antibiotics.
- Antimycobacterial Activity : Demonstrated efficacy against Mycobacterium tuberculosis, making it a candidate for tuberculosis treatment.
- Antitumor Activity : Inhibits the growth of cancer cell lines, including HeLa and A549, with observed IC50 values indicating significant antiproliferative effects.
- Anti-inflammatory Effects : Exhibits properties that may reduce inflammation, contributing to its potential use in treating inflammatory diseases.
- Antiviral Activity : Shows promise in inhibiting viral replication in certain studies.
The biological activity of this compound is attributed to its ability to interact with various biological targets:
- Enzyme Inhibition : The compound can inhibit enzymes critical for bacterial and cancer cell survival.
- DNA Interaction : It may interact with DNA or RNA, disrupting replication processes in pathogens and cancer cells.
- Receptor Binding : The imidazole ring can participate in hydrogen bonding and coordination with metal ions, influencing receptor activity and cellular signaling pathways.
Structure-Activity Relationship (SAR)
The structure-activity relationship studies indicate that modifications to the imidazole or pyridine rings can significantly affect the biological activity of derivatives. For example:
Modification | Effect on Activity | IC50 Value (µM) |
---|---|---|
Hydroxyl group addition | Increases antiproliferative activity | 0.021 (against PC3) |
Bromine substitution | Enhances antibacterial properties | Not specified |
Methylation of nitrogen | Reduces enzyme inhibition | Not specified |
Case Studies
-
Antitumor Efficacy :
A study evaluated the antiproliferative effects of this compound derivatives on various cancer cell lines. Results demonstrated significant inhibition of cell growth, particularly in HeLa cells, with an IC50 value as low as 0.021 µM for specific derivatives . -
Antimicrobial Studies :
Research has shown that compounds similar to this compound exhibit potent activity against both Gram-positive and Gram-negative bacteria. The mechanism involves disruption of bacterial cell wall synthesis and interference with metabolic pathways . -
Inflammation Models :
In vivo studies have indicated that the compound can reduce markers of inflammation in animal models, suggesting its potential utility in treating inflammatory diseases .
Pharmacokinetics
The pharmacokinetic profile of this compound suggests good solubility in polar solvents, which enhances its bioavailability. Studies indicate that it is rapidly absorbed and metabolized, with a favorable half-life for therapeutic applications.
Q & A
Basic Research Questions
Q. What are common synthetic routes for 3-(1H-imidazol-2-yl)pyridine and its metal complexes?
this compound (HIPy) is typically synthesized via solvothermal methods or reflux reactions. For example, HIPy-based copper(I) polymers are prepared by reacting HIPy with CuI under solvothermal conditions, yielding coordination polymers with unique structural motifs . Cadmium(II) complexes are synthesized by refluxing HIPy with Cd(NO₃)₂ in ethanol, forming mononuclear complexes with octahedral geometry . Key steps include ligand purification (e.g., recrystallization) and characterization via elemental analysis and spectroscopic methods.
Q. How is the crystal structure of HIPy-based complexes determined?
Single-crystal X-ray diffraction (SCXRD) is the gold standard. For example, the triclinic crystal system of [Cu₂I₂(HIPy)₂]ₙ was resolved using SHELX software , with unit cell parameters:
Parameter | Value |
---|---|
a | 8.141 Å |
b | 8.306 Å |
c | 8.816 Å |
α, β, γ | 114.7°, 102.0°, 108.3° |
V | 473.1 ų |
Data collection involves high-resolution detectors and refinement using programs like SHELXL . |
Q. What spectroscopic techniques are used to characterize HIPy derivatives?
- FTIR : Identifies functional groups (e.g., N–H stretching at ~3100 cm⁻¹ in imidazole rings) .
- ESI-MS : Confirms molecular ion peaks (e.g., [Eu(dbm)₃(HIPy)]⁺ at m/z 1065) .
- Photoluminescence : Evaluates emission properties (e.g., Eu(III) complexes exhibit red emission at 612 nm) .
Advanced Research Questions
Q. How can molecular docking predict HIPy derivatives as kinase inhibitors?
HIPy derivatives like 2-(1H-imidazol-2-yl)pyridine analogs are screened against targets (e.g., V600E-BRAF kinase) using:
Ligand Preparation : 2D structures drawn in ChemDraw, converted to 3D via Spartan 14, and optimized with MM2 force fields .
Docking Software : Molegro Virtual Docker (MVD) evaluates binding scores. For example, Compound 14 showed a docking score of −124.079 vs. −116.174 for vemurafenib .
Key Interactions : Hydrogen bonds with residues like CYS532 and hydrophobic interactions with TRP531 .
Table 1 : Top HIPy derivatives as V600E-BRAF inhibitors
Compound | Docking Score | Key Interactions |
---|---|---|
14 | −124.079 | CYS532, ASP594 |
30 | −122.290 | PHE583, TRP531 |
Vemurafenib | −116.174 | Reference ligand |
Q. How do ligand geometry and coordination modes affect metal-organic frameworks (MOFs)?
HIPy’s flexibility allows diverse coordination modes. For example:
- Monodentate : HIPy binds Cd(II) via pyridyl-N in [Cd(HIPy)₂(NO₃)₂(H₂O)₂], forming an N₂O₄ octahedron .
- Bridging : In Cu(I) polymers, HIPy links Cu centers via µ-iodide bridges, creating 1D chains .
Distorted geometries (e.g., square antiprismatic Eu(III) complexes) impact photophysical properties .
Q. What computational methods validate HIPy’s drug-likeness and pharmacokinetics?
- Lipinski’s Rule : Assess oral bioavailability (e.g., molecular weight <500, LogP <5). HIPy derivatives comply with ≤1 violation .
- ADMET Prediction : Tools like SwissADME predict absorption (e.g., Caco-2 permeability) and toxicity (e.g., hepatotoxicity risk). Compounds 14 and 30 show favorable profiles .
Q. How are HIPy-based MOFs optimized for functional applications?
- Porosity Tuning : Adjusting synthetic conditions (e.g., solvent polarity) modifies pore size.
- Stability Tests : Thermogravimetric analysis (TGA) reveals decomposition temperatures (e.g., [Eu(dbm)₃(HIPy)] stable up to 250°C) .
- Photoluminescence : Ligand-to-metal charge transfer (LMCT) enhances emission intensity in lanthanide complexes .
Q. Methodological Considerations
- Crystallography : Use SHELX for refinement and WinGX for data visualization .
- Docking Validation : Cross-check docking poses with experimental IC₅₀ values or mutagenesis studies.
- Synthetic Reproducibility : Control reflux time (e.g., 5 hours for Cd(II) complexes ) and stoichiometry to avoid byproducts.
Properties
IUPAC Name |
3-(1H-imidazol-2-yl)pyridine | |
---|---|---|
Source | PubChem | |
URL | https://pubchem.ncbi.nlm.nih.gov | |
Description | Data deposited in or computed by PubChem | |
InChI |
InChI=1S/C8H7N3/c1-2-7(6-9-3-1)8-10-4-5-11-8/h1-6H,(H,10,11) | |
Source | PubChem | |
URL | https://pubchem.ncbi.nlm.nih.gov | |
Description | Data deposited in or computed by PubChem | |
InChI Key |
ZQZJULZPQACTES-UHFFFAOYSA-N | |
Source | PubChem | |
URL | https://pubchem.ncbi.nlm.nih.gov | |
Description | Data deposited in or computed by PubChem | |
Canonical SMILES |
C1=CC(=CN=C1)C2=NC=CN2 | |
Source | PubChem | |
URL | https://pubchem.ncbi.nlm.nih.gov | |
Description | Data deposited in or computed by PubChem | |
Molecular Formula |
C8H7N3 | |
Source | PubChem | |
URL | https://pubchem.ncbi.nlm.nih.gov | |
Description | Data deposited in or computed by PubChem | |
DSSTOX Substance ID |
DTXSID40496206 | |
Record name | 3-(1H-Imidazol-2-yl)pyridine | |
Source | EPA DSSTox | |
URL | https://comptox.epa.gov/dashboard/DTXSID40496206 | |
Description | DSSTox provides a high quality public chemistry resource for supporting improved predictive toxicology. | |
Molecular Weight |
145.16 g/mol | |
Source | PubChem | |
URL | https://pubchem.ncbi.nlm.nih.gov | |
Description | Data deposited in or computed by PubChem | |
CAS No. |
13570-00-8 | |
Record name | 3-(1H-Imidazol-2-yl)pyridine | |
Source | EPA DSSTox | |
URL | https://comptox.epa.gov/dashboard/DTXSID40496206 | |
Description | DSSTox provides a high quality public chemistry resource for supporting improved predictive toxicology. | |
Synthesis routes and methods
Procedure details
Retrosynthesis Analysis
AI-Powered Synthesis Planning: Our tool employs the Template_relevance Pistachio, Template_relevance Bkms_metabolic, Template_relevance Pistachio_ringbreaker, Template_relevance Reaxys, Template_relevance Reaxys_biocatalysis model, leveraging a vast database of chemical reactions to predict feasible synthetic routes.
One-Step Synthesis Focus: Specifically designed for one-step synthesis, it provides concise and direct routes for your target compounds, streamlining the synthesis process.
Accurate Predictions: Utilizing the extensive PISTACHIO, BKMS_METABOLIC, PISTACHIO_RINGBREAKER, REAXYS, REAXYS_BIOCATALYSIS database, our tool offers high-accuracy predictions, reflecting the latest in chemical research and data.
Strategy Settings
Precursor scoring | Relevance Heuristic |
---|---|
Min. plausibility | 0.01 |
Model | Template_relevance |
Template Set | Pistachio/Bkms_metabolic/Pistachio_ringbreaker/Reaxys/Reaxys_biocatalysis |
Top-N result to add to graph | 6 |
Feasible Synthetic Routes
Disclaimer and Information on In-Vitro Research Products
Please be aware that all articles and product information presented on BenchChem are intended solely for informational purposes. The products available for purchase on BenchChem are specifically designed for in-vitro studies, which are conducted outside of living organisms. In-vitro studies, derived from the Latin term "in glass," involve experiments performed in controlled laboratory settings using cells or tissues. It is important to note that these products are not categorized as medicines or drugs, and they have not received approval from the FDA for the prevention, treatment, or cure of any medical condition, ailment, or disease. We must emphasize that any form of bodily introduction of these products into humans or animals is strictly prohibited by law. It is essential to adhere to these guidelines to ensure compliance with legal and ethical standards in research and experimentation.