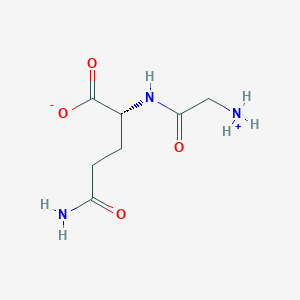
Glycyl-d-glutamine
Overview
Description
Glycyl-d-glutamine is a dipeptide composed of glycine and d-glutamine. It is known for its stability and solubility, making it a valuable compound in various scientific and industrial applications. This compound is particularly significant in the field of nutrition and medicine due to its role in enhancing gut health and supporting immune function.
Preparation Methods
Synthetic Routes and Reaction Conditions: The preparation of glycyl-d-glutamine typically involves the protection of the N-terminal of glycine using tert-butoxycarbonyl (Boc) anhydride, followed by condensation with d-glutamine using chloroformate through a mixed anhydride method. The final step involves deprotection using trifluoroacetic acid to obtain the crude product, which is then purified through recrystallization .
Industrial Production Methods: Industrial production methods for this compound focus on optimizing reaction conditions to ensure high yield and purity. One method involves mixing d-glutamine with carbonate water solution, followed by the addition of phthalimide acetyl chloride. The resulting product undergoes hydrazinolysis with hydrazine hydrate to yield this compound . These methods are designed to be environmentally friendly and cost-effective, avoiding the use of toxic solvents.
Chemical Reactions Analysis
Types of Reactions: Glycyl-d-glutamine undergoes various chemical reactions, including deamidation, hydrolysis, and condensation. Deamidation involves the hydrolysis of amide bonds, producing ammonia and the corresponding acidic amino acids .
Common Reagents and Conditions:
Deamidation: Catalyzed by enzymes such as glutaminases, which hydrolyze the gamma-amido bond of d-glutamine residues.
Hydrolysis: Typically occurs under acidic or basic conditions, leading to the breakdown of the dipeptide into its constituent amino acids.
Condensation: Involves the use of chloroformate and Boc anhydride for the protection and activation of amino acids.
Major Products: The major products formed from these reactions include ammonia, glutamic acid, and glycine. These products are essential for various metabolic processes and have significant biological functions.
Scientific Research Applications
Glycyl-d-glutamine has a wide range of applications in scientific research:
Chemistry: Used as a stable dipeptide for studying peptide synthesis and degradation.
Industry: Utilized in the food industry to enhance the flavor and functional properties of proteins.
Mechanism of Action
The mechanism of action of glycyl-d-glutamine involves its hydrolysis into glycine and d-glutamine, which are then utilized in various metabolic pathways. Glycine acts as a neurotransmitter and a precursor for the synthesis of proteins and other biomolecules. d-Glutamine is involved in nitrogen transport and serves as an energy source for rapidly dividing cells . The compound also modulates the expression of genes involved in cell metabolism and immune response .
Comparison with Similar Compounds
Alanyl-glutamine: Another dipeptide used as a glutamine substitute, known for its high solubility and stability.
Glutamic acid derivatives: Compounds that modify the behavior of glutamine and glutamic acid in cancer cells, offering potential therapeutic benefits.
Uniqueness: Glycyl-d-glutamine is unique due to its specific combination of glycine and d-glutamine, which provides enhanced stability and solubility compared to other dipeptides. Its ability to modulate gut microbiota and support immune function makes it particularly valuable in medical and nutritional applications .
Properties
IUPAC Name |
(2R)-5-amino-2-[(2-aminoacetyl)amino]-5-oxopentanoic acid | |
---|---|---|
Source | PubChem | |
URL | https://pubchem.ncbi.nlm.nih.gov | |
Description | Data deposited in or computed by PubChem | |
InChI |
InChI=1S/C7H13N3O4/c8-3-6(12)10-4(7(13)14)1-2-5(9)11/h4H,1-3,8H2,(H2,9,11)(H,10,12)(H,13,14)/t4-/m1/s1 | |
Source | PubChem | |
URL | https://pubchem.ncbi.nlm.nih.gov | |
Description | Data deposited in or computed by PubChem | |
InChI Key |
PNMUAGGSDZXTHX-SCSAIBSYSA-N | |
Source | PubChem | |
URL | https://pubchem.ncbi.nlm.nih.gov | |
Description | Data deposited in or computed by PubChem | |
Canonical SMILES |
C(CC(=O)N)C(C(=O)O)NC(=O)CN | |
Source | PubChem | |
URL | https://pubchem.ncbi.nlm.nih.gov | |
Description | Data deposited in or computed by PubChem | |
Isomeric SMILES |
C(CC(=O)N)[C@H](C(=O)O)NC(=O)CN | |
Source | PubChem | |
URL | https://pubchem.ncbi.nlm.nih.gov | |
Description | Data deposited in or computed by PubChem | |
Molecular Formula |
C7H13N3O4 | |
Source | PubChem | |
URL | https://pubchem.ncbi.nlm.nih.gov | |
Description | Data deposited in or computed by PubChem | |
Molecular Weight |
203.20 g/mol | |
Source | PubChem | |
URL | https://pubchem.ncbi.nlm.nih.gov | |
Description | Data deposited in or computed by PubChem | |
Retrosynthesis Analysis
AI-Powered Synthesis Planning: Our tool employs the Template_relevance Pistachio, Template_relevance Bkms_metabolic, Template_relevance Pistachio_ringbreaker, Template_relevance Reaxys, Template_relevance Reaxys_biocatalysis model, leveraging a vast database of chemical reactions to predict feasible synthetic routes.
One-Step Synthesis Focus: Specifically designed for one-step synthesis, it provides concise and direct routes for your target compounds, streamlining the synthesis process.
Accurate Predictions: Utilizing the extensive PISTACHIO, BKMS_METABOLIC, PISTACHIO_RINGBREAKER, REAXYS, REAXYS_BIOCATALYSIS database, our tool offers high-accuracy predictions, reflecting the latest in chemical research and data.
Strategy Settings
Precursor scoring | Relevance Heuristic |
---|---|
Min. plausibility | 0.01 |
Model | Template_relevance |
Template Set | Pistachio/Bkms_metabolic/Pistachio_ringbreaker/Reaxys/Reaxys_biocatalysis |
Top-N result to add to graph | 6 |
Feasible Synthetic Routes
Q1: How does glycyl-L-glutamine interact with cardiac cells and what are the downstream effects?
A1: While the exact mechanism of interaction remains unclear, research suggests that glycyl-L-glutamine exerts a trophic effect on developing rat heart cells. [] This means it supports the growth and survival of these cells, specifically influencing the cholinergic system, which is crucial for communication within the heart.
Q2: Does glycyl-L-glutamine affect the activity of acetylcholinesterase in cardiac cells?
A2: Interestingly, while glycyl-L-glutamine influences the expression of specific AChE molecular forms, it does not appear to alter the overall enzymatic activity of AChE in cultured post-natal ventricular myocytes. [] This suggests that its influence might be more focused on regulating the structural organization and localization of AChE, rather than directly modulating its catalytic activity.
Disclaimer and Information on In-Vitro Research Products
Please be aware that all articles and product information presented on BenchChem are intended solely for informational purposes. The products available for purchase on BenchChem are specifically designed for in-vitro studies, which are conducted outside of living organisms. In-vitro studies, derived from the Latin term "in glass," involve experiments performed in controlled laboratory settings using cells or tissues. It is important to note that these products are not categorized as medicines or drugs, and they have not received approval from the FDA for the prevention, treatment, or cure of any medical condition, ailment, or disease. We must emphasize that any form of bodily introduction of these products into humans or animals is strictly prohibited by law. It is essential to adhere to these guidelines to ensure compliance with legal and ethical standards in research and experimentation.