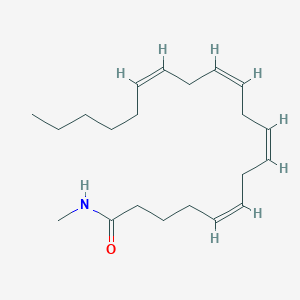
Arachidonoyl-N-methyl amide
Overview
Description
Arachidonoyl-N-methyl amide (CAS Number 156910-29-1) is an analog of : this compound (CAS Number: 156910-29-1) is an analog of anandamide (AEA), an endogenous cannabinoid. It binds to both the central cannabinoid receptor (CB1) and the peripheral cannabinoid receptor (CB2). The biological actions of AEA are terminated by cellular uptake and hydrolysis of the amide bond by the enzyme fatty acid amide hydrolase .
Mechanism of Action
Target of Action
Arachidonoyl-N-methyl amide, an analog of anandamide, primarily targets the human central cannabinoid (CB1) receptor . The CB1 receptor is a part of the endocannabinoid system, which plays a crucial role in regulating various physiological processes such as pain sensation, mood, and memory.
Mode of Action
this compound interacts with the CB1 receptor, displaying a binding affinity with a Ki of 60 nM . This interaction leads to the inhibition of rat glial gap junction cell-cell communication .
Biochemical Pathways
The compound is part of the fatty acid amide family, which is structurally related to the endocannabinoid anandamide . The biological actions of anandamide are terminated by cellular uptake and hydrolysis of the amide bond by the enzyme fatty acid amide hydrolase . This suggests that this compound might follow a similar pathway.
Pharmacokinetics
It is known that the compound is a lipophilic substance, which suggests that it can readily cross the blood-brain barrier and interact with receptors in both the central and peripheral nervous systems .
Result of Action
The interaction of this compound with the CB1 receptor leads to the inhibition of rat glial gap junction cell-cell communication . This could potentially influence various physiological processes regulated by the endocannabinoid system, such as pain sensation, mood, and memory.
Biochemical Analysis
Biochemical Properties
Arachidonoyl-N-methyl amide interacts with various biomolecules, including enzymes and proteins . It binds to the human central cannabinoid (CB1) receptor with a Ki of 60 nM . The biological actions of this compound are terminated by cellular uptake and hydrolysis of the amide bond by the enzyme fatty acid amide hydrolase .
Cellular Effects
This compound influences cell function by interacting with cell signaling pathways . It binds to cannabinoid receptors, which may impact gene expression and cellular metabolism .
Molecular Mechanism
This compound exerts its effects at the molecular level through binding interactions with biomolecules . It binds to the CB1 receptor, and this interaction can lead to changes in gene expression .
Temporal Effects in Laboratory Settings
The effects of this compound can change over time in laboratory settings
Dosage Effects in Animal Models
The effects of this compound can vary with different dosages in animal models
Metabolic Pathways
This compound is involved in metabolic pathways . It is metabolized by the enzyme fatty acid amide hydrolase
Transport and Distribution
This compound is transported and distributed within cells and tissues
Preparation Methods
Synthetic Routes:: Arachidonoyl-N-methyl amide can be synthesized through organic chemistry methods. While specific synthetic routes may vary, the general approach involves the condensation of arachidonic acid with methylamine. The reaction typically occurs under controlled conditions, such as using appropriate solvents and catalysts.
Industrial Production:: Although not widely produced industrially, this compound can be synthesized on a laboratory scale for research purposes.
Chemical Reactions Analysis
Reactions:: Arachidonoyl-N-methyl amide can undergo various chemical reactions, including oxidation, reduction, and substitution. detailed studies on its reactivity are limited.
Common Reagents and Conditions::- Oxidation : Oxidizing agents like potassium permanganate (KMnO4) or chromic acid (H2CrO4) can be used.
- Reduction : Reducing agents such as lithium aluminum hydride (LiAlH4) or sodium borohydride (NaBH4) may reduce the amide bond.
- Substitution : Nucleophilic substitution reactions can occur with appropriate reagents.
Major Products:: The major products formed from these reactions would include derivatives of this compound with modified functional groups.
Scientific Research Applications
Arachidonoyl-N-methyl amide has diverse applications:
- Chemistry : It serves as a model compound for studying lipid metabolism and cannabinoid receptors.
- Biology : Researchers explore its role in endocannabinoid signaling pathways.
- Medicine : Investigations focus on its potential therapeutic effects, although clinical applications remain speculative.
- Industry : Limited industrial applications exist due to its specialized nature.
Comparison with Similar Compounds
Arachidonoyl-N-methyl amide’s uniqueness lies in its structural similarity to anandamide. Other related compounds include anandamide itself and other endocannabinoids.
Properties
IUPAC Name |
(5Z,8Z,11Z,14Z)-N-methylicosa-5,8,11,14-tetraenamide | |
---|---|---|
Source | PubChem | |
URL | https://pubchem.ncbi.nlm.nih.gov | |
Description | Data deposited in or computed by PubChem | |
InChI |
InChI=1S/C21H35NO/c1-3-4-5-6-7-8-9-10-11-12-13-14-15-16-17-18-19-20-21(23)22-2/h7-8,10-11,13-14,16-17H,3-6,9,12,15,18-20H2,1-2H3,(H,22,23)/b8-7-,11-10-,14-13-,17-16- | |
Source | PubChem | |
URL | https://pubchem.ncbi.nlm.nih.gov | |
Description | Data deposited in or computed by PubChem | |
InChI Key |
HTAWYBRCXMQDBL-ZKWNWVNESA-N | |
Source | PubChem | |
URL | https://pubchem.ncbi.nlm.nih.gov | |
Description | Data deposited in or computed by PubChem | |
Canonical SMILES |
CCCCCC=CCC=CCC=CCC=CCCCC(=O)NC | |
Source | PubChem | |
URL | https://pubchem.ncbi.nlm.nih.gov | |
Description | Data deposited in or computed by PubChem | |
Isomeric SMILES |
CCCCC/C=C\C/C=C\C/C=C\C/C=C\CCCC(=O)NC | |
Source | PubChem | |
URL | https://pubchem.ncbi.nlm.nih.gov | |
Description | Data deposited in or computed by PubChem | |
Molecular Formula |
C21H35NO | |
Source | PubChem | |
URL | https://pubchem.ncbi.nlm.nih.gov | |
Description | Data deposited in or computed by PubChem | |
Molecular Weight |
317.5 g/mol | |
Source | PubChem | |
URL | https://pubchem.ncbi.nlm.nih.gov | |
Description | Data deposited in or computed by PubChem | |
Retrosynthesis Analysis
AI-Powered Synthesis Planning: Our tool employs the Template_relevance Pistachio, Template_relevance Bkms_metabolic, Template_relevance Pistachio_ringbreaker, Template_relevance Reaxys, Template_relevance Reaxys_biocatalysis model, leveraging a vast database of chemical reactions to predict feasible synthetic routes.
One-Step Synthesis Focus: Specifically designed for one-step synthesis, it provides concise and direct routes for your target compounds, streamlining the synthesis process.
Accurate Predictions: Utilizing the extensive PISTACHIO, BKMS_METABOLIC, PISTACHIO_RINGBREAKER, REAXYS, REAXYS_BIOCATALYSIS database, our tool offers high-accuracy predictions, reflecting the latest in chemical research and data.
Strategy Settings
Precursor scoring | Relevance Heuristic |
---|---|
Min. plausibility | 0.01 |
Model | Template_relevance |
Template Set | Pistachio/Bkms_metabolic/Pistachio_ringbreaker/Reaxys/Reaxys_biocatalysis |
Top-N result to add to graph | 6 |
Feasible Synthetic Routes
Q1: The study identifies N-methyl arachidonoyl amine as a potential biomarker for distinguishing prostate cancer from benign prostatic hyperplasia. What is the significance of its negative correlation with lipid profiles like total cholesterol, LDL-C, and Apo-B in prostate cancer patients?
A1: The negative correlation between N-methyl arachidonoyl amine and certain lipid profiles (total cholesterol, LDL-C, and Apo-B) specifically in prostate cancer patients [] presents an intriguing observation. While the study itself doesn't delve into the underlying mechanisms of this correlation, it suggests a potential interplay between N-methyl arachidonoyl amine and lipid metabolism pathways that are distinctly altered in prostate cancer. Further research is crucial to understand if this altered lipid metabolism is a driver or a consequence of the disease and if N-methyl arachidonoyl amine plays a direct or indirect role in this process. This knowledge could be crucial for developing novel diagnostic or therapeutic strategies targeting lipid metabolism in prostate cancer.
Disclaimer and Information on In-Vitro Research Products
Please be aware that all articles and product information presented on BenchChem are intended solely for informational purposes. The products available for purchase on BenchChem are specifically designed for in-vitro studies, which are conducted outside of living organisms. In-vitro studies, derived from the Latin term "in glass," involve experiments performed in controlled laboratory settings using cells or tissues. It is important to note that these products are not categorized as medicines or drugs, and they have not received approval from the FDA for the prevention, treatment, or cure of any medical condition, ailment, or disease. We must emphasize that any form of bodily introduction of these products into humans or animals is strictly prohibited by law. It is essential to adhere to these guidelines to ensure compliance with legal and ethical standards in research and experimentation.