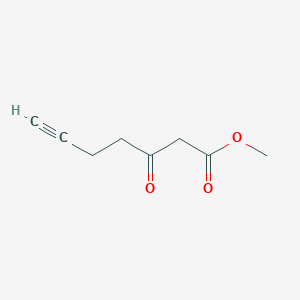
Methyl 3-oxohept-6-ynoate
Overview
Description
Methyl 3-oxohept-6-ynoate is an organic compound with the molecular formula C8H10O3. It is a colorless to yellow liquid or viscous liquid that is used in various chemical reactions and research applications. The compound is characterized by the presence of a keto group at the third position and an alkyne group at the sixth position of the heptane chain.
Preparation Methods
Synthetic Routes and Reaction Conditions: Methyl 3-oxohept-6-ynoate can be synthesized through a multi-step reaction process. One common method involves the use of 4-pentynoic acid as a starting material. The synthesis typically involves the following steps:
Dicyclohexylcarbodiimide (DCC) and 4-(N,N-dimethylamino)pyridine (DMAP) in dichloromethane (CH2Cl2): This step is carried out at ambient temperature for 24 hours.
Heating: The reaction mixture is then heated to complete the synthesis.
Industrial Production Methods:
Chemical Reactions Analysis
Types of Reactions: Methyl 3-oxohept-6-ynoate undergoes various types of chemical reactions, including:
Oxidation: The compound can be oxidized to form corresponding carboxylic acids or other oxidized derivatives.
Reduction: Reduction reactions can convert the keto group to a hydroxyl group, forming alcohols.
Substitution: The alkyne group can participate in substitution reactions, leading to the formation of various substituted derivatives.
Common Reagents and Conditions:
Oxidation: Common oxidizing agents include potassium permanganate (KMnO4) and chromium trioxide (CrO3).
Reduction: Reducing agents such as sodium borohydride (NaBH4) or lithium aluminum hydride (LiAlH4) are commonly used.
Substitution: Reagents like halogens (e.g., bromine) or nucleophiles (e.g., amines) can be used for substitution reactions.
Major Products Formed:
Oxidation: Carboxylic acids or other oxidized derivatives.
Reduction: Alcohols.
Substitution: Substituted derivatives with various functional groups
Scientific Research Applications
Methyl 3-oxohept-6-ynoate has several scientific research applications, including:
Chemistry: It is used as an intermediate in the synthesis of more complex organic molecules.
Biology: The compound can be used in studies involving enzyme-catalyzed reactions and metabolic pathways.
Medicine: Research into potential pharmaceutical applications, including the development of new drugs.
Industry: Utilized in the production of fine chemicals and specialty materials
Mechanism of Action
The mechanism of action of methyl 3-oxohept-6-ynoate involves its interaction with various molecular targets and pathways. The keto group and alkyne group are reactive sites that can participate in various chemical reactions. The compound can act as a substrate for enzymes, leading to the formation of different products through enzymatic catalysis. The specific pathways and molecular targets depend on the context of the reaction and the biological system involved .
Comparison with Similar Compounds
Methyl 3-oxohept-6-ynoate can be compared with other similar compounds, such as:
Methyl 3-oxohept-6-enoate: This compound has a similar structure but contains a double bond instead of a triple bond.
Methyl 3-oxooct-6-ynoate: This compound has an additional carbon in the chain, making it an octane derivative.
Methyl 3-oxohex-6-ynoate: This compound has one less carbon in the chain, making it a hexane derivative.
Uniqueness: this compound is unique due to the presence of both a keto group and an alkyne group in its structure. This combination of functional groups allows it to participate in a wide range of chemical reactions, making it a versatile compound for research and industrial applications .
Properties
IUPAC Name |
methyl 3-oxohept-6-ynoate | |
---|---|---|
Source | PubChem | |
URL | https://pubchem.ncbi.nlm.nih.gov | |
Description | Data deposited in or computed by PubChem | |
InChI |
InChI=1S/C8H10O3/c1-3-4-5-7(9)6-8(10)11-2/h1H,4-6H2,2H3 | |
Source | PubChem | |
URL | https://pubchem.ncbi.nlm.nih.gov | |
Description | Data deposited in or computed by PubChem | |
InChI Key |
HSZWYTRZAPFGTG-UHFFFAOYSA-N | |
Source | PubChem | |
URL | https://pubchem.ncbi.nlm.nih.gov | |
Description | Data deposited in or computed by PubChem | |
Canonical SMILES |
COC(=O)CC(=O)CCC#C | |
Source | PubChem | |
URL | https://pubchem.ncbi.nlm.nih.gov | |
Description | Data deposited in or computed by PubChem | |
Molecular Formula |
C8H10O3 | |
Source | PubChem | |
URL | https://pubchem.ncbi.nlm.nih.gov | |
Description | Data deposited in or computed by PubChem | |
DSSTOX Substance ID |
DTXSID50438470 | |
Record name | 6-Heptynoic acid, 3-oxo-, methyl ester | |
Source | EPA DSSTox | |
URL | https://comptox.epa.gov/dashboard/DTXSID50438470 | |
Description | DSSTox provides a high quality public chemistry resource for supporting improved predictive toxicology. | |
Molecular Weight |
154.16 g/mol | |
Source | PubChem | |
URL | https://pubchem.ncbi.nlm.nih.gov | |
Description | Data deposited in or computed by PubChem | |
CAS No. |
100330-50-5 | |
Record name | 6-Heptynoic acid, 3-oxo-, methyl ester | |
Source | EPA DSSTox | |
URL | https://comptox.epa.gov/dashboard/DTXSID50438470 | |
Description | DSSTox provides a high quality public chemistry resource for supporting improved predictive toxicology. | |
Retrosynthesis Analysis
AI-Powered Synthesis Planning: Our tool employs the Template_relevance Pistachio, Template_relevance Bkms_metabolic, Template_relevance Pistachio_ringbreaker, Template_relevance Reaxys, Template_relevance Reaxys_biocatalysis model, leveraging a vast database of chemical reactions to predict feasible synthetic routes.
One-Step Synthesis Focus: Specifically designed for one-step synthesis, it provides concise and direct routes for your target compounds, streamlining the synthesis process.
Accurate Predictions: Utilizing the extensive PISTACHIO, BKMS_METABOLIC, PISTACHIO_RINGBREAKER, REAXYS, REAXYS_BIOCATALYSIS database, our tool offers high-accuracy predictions, reflecting the latest in chemical research and data.
Strategy Settings
Precursor scoring | Relevance Heuristic |
---|---|
Min. plausibility | 0.01 |
Model | Template_relevance |
Template Set | Pistachio/Bkms_metabolic/Pistachio_ringbreaker/Reaxys/Reaxys_biocatalysis |
Top-N result to add to graph | 6 |
Feasible Synthetic Routes
Disclaimer and Information on In-Vitro Research Products
Please be aware that all articles and product information presented on BenchChem are intended solely for informational purposes. The products available for purchase on BenchChem are specifically designed for in-vitro studies, which are conducted outside of living organisms. In-vitro studies, derived from the Latin term "in glass," involve experiments performed in controlled laboratory settings using cells or tissues. It is important to note that these products are not categorized as medicines or drugs, and they have not received approval from the FDA for the prevention, treatment, or cure of any medical condition, ailment, or disease. We must emphasize that any form of bodily introduction of these products into humans or animals is strictly prohibited by law. It is essential to adhere to these guidelines to ensure compliance with legal and ethical standards in research and experimentation.