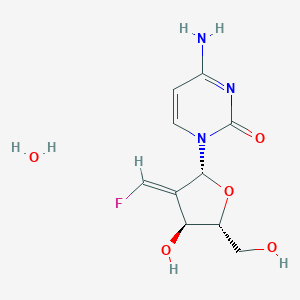
Tezacitabine monohydrate
Overview
Description
Preparation Methods
Synthetic Routes and Reaction Conditions: The synthesis of tezacitabine involves several steps:
Protection of Cytidine: Cytidine is protected using 1,3-dichloro-1,1,3,3-tetraisopropyldisiloxane in pyridine to yield a fully protected cytidine derivative.
Fluoromethylation: The 2’-deoxy-2’-oxo derivative is reacted with fluoromethyl phenyl sulfone using diethyl chlorophosphate and lithium hexamethyldisylazide in THF to produce a fluorovinyl sulfone.
Separation of Isomers: The fluorovinyl sulfone is separated into (E) and (Z) isomers by flash chromatography.
Industrial Production Methods: Industrial production methods for tezacitabine focus on optimizing the yield and purity of the compound. These methods often involve large-scale synthesis using the same reaction steps as described above, with additional purification steps to ensure the stability and efficacy of the final product .
Chemical Reactions Analysis
Types of Reactions: Tezacitabine undergoes several types of chemical reactions, including:
Phosphorylation: Phosphorylated by cellular kinases to form active diphosphate and triphosphate metabolites.
Inhibition of Enzymes: The diphosphate form irreversibly inhibits ribonucleotide reductase, while the triphosphate form acts as a substrate for DNA polymerase.
Common Reagents and Conditions:
Phosphorylation: Cellular kinases are the primary reagents involved in the phosphorylation of tezacitabine.
Inhibition of Enzymes: The inhibition of ribonucleotide reductase and DNA polymerase occurs under physiological conditions within the cell.
Major Products Formed:
Diphosphate and Triphosphate Metabolites: These active metabolites are formed through phosphorylation and are responsible for the compound’s antineoplastic activity.
Scientific Research Applications
Tezacitabine has a wide range of scientific research applications, including:
Chemistry: Used as a synthetic purine nucleoside analogue in various chemical studies.
Biology: Investigated for its role in inhibiting DNA synthesis and inducing apoptosis in tumor cells.
Medicine: Explored as a potential treatment for various cancers, including colorectal cancer, lung cancer, leukemia, and gastric cancer
Mechanism of Action
Tezacitabine exerts its effects through a dual mechanism of action:
Inhibition of Ribonucleotide Reductase: The diphosphate form of tezacitabine binds to and irreversibly inhibits ribonucleotide reductase, leading to the inhibition of DNA synthesis in tumor cells.
DNA Chain Termination: The triphosphate form of tezacitabine acts as a substrate for DNA polymerase, resulting in the incorporation into DNA and subsequent chain termination.
Molecular Targets and Pathways:
Comparison with Similar Compounds
Properties
IUPAC Name |
4-amino-1-[(2R,3E,4S,5R)-3-(fluoromethylidene)-4-hydroxy-5-(hydroxymethyl)oxolan-2-yl]pyrimidin-2-one | |
---|---|---|
Source | PubChem | |
URL | https://pubchem.ncbi.nlm.nih.gov | |
Description | Data deposited in or computed by PubChem | |
InChI |
InChI=1S/C10H12FN3O4/c11-3-5-8(16)6(4-15)18-9(5)14-2-1-7(12)13-10(14)17/h1-3,6,8-9,15-16H,4H2,(H2,12,13,17)/b5-3+/t6-,8+,9-/m1/s1 | |
Source | PubChem | |
URL | https://pubchem.ncbi.nlm.nih.gov | |
Description | Data deposited in or computed by PubChem | |
InChI Key |
GFFXZLZWLOBBLO-ASKVSEFXSA-N | |
Source | PubChem | |
URL | https://pubchem.ncbi.nlm.nih.gov | |
Description | Data deposited in or computed by PubChem | |
Canonical SMILES |
C1=CN(C(=O)N=C1N)C2C(=CF)C(C(O2)CO)O | |
Source | PubChem | |
URL | https://pubchem.ncbi.nlm.nih.gov | |
Description | Data deposited in or computed by PubChem | |
Isomeric SMILES |
C1=CN(C(=O)N=C1N)[C@H]2/C(=C/F)/[C@@H]([C@H](O2)CO)O | |
Source | PubChem | |
URL | https://pubchem.ncbi.nlm.nih.gov | |
Description | Data deposited in or computed by PubChem | |
Molecular Formula |
C10H12FN3O4 | |
Source | PubChem | |
URL | https://pubchem.ncbi.nlm.nih.gov | |
Description | Data deposited in or computed by PubChem | |
DSSTOX Substance ID |
DTXSID10156446 | |
Record name | Tezacitabine | |
Source | EPA DSSTox | |
URL | https://comptox.epa.gov/dashboard/DTXSID10156446 | |
Description | DSSTox provides a high quality public chemistry resource for supporting improved predictive toxicology. | |
Molecular Weight |
257.22 g/mol | |
Source | PubChem | |
URL | https://pubchem.ncbi.nlm.nih.gov | |
Description | Data deposited in or computed by PubChem | |
Mechanism of Action |
Phosphorylated by cellular kinases, tezacitabine is converted into its active diphosphate and triphosphate metabolites. Tezacitabine diphosphate binds to and irreversibly inhibits the activity of the enzyme ribonucleotide reductase (RNR), which may result in the inhibition of DNA synthesis in tumor cells and tumor cell apoptosis. Tezacitabine triphosphate acts as a substrate for DNA polymerase, further compromising DNA replication. This agent is relatively resistant to metabolic deactivation by cytidine deaminase. RNR catalyzes the conversion of ribonucleoside 5'-diphosphates to deoxyribonucleoside 5'-diphosphates necessary for DNA synthesis and is overexpressed in many tumor types. | |
Record name | Tezacitabine | |
Source | DrugBank | |
URL | https://www.drugbank.ca/drugs/DB06433 | |
Description | The DrugBank database is a unique bioinformatics and cheminformatics resource that combines detailed drug (i.e. chemical, pharmacological and pharmaceutical) data with comprehensive drug target (i.e. sequence, structure, and pathway) information. | |
Explanation | Creative Common's Attribution-NonCommercial 4.0 International License (http://creativecommons.org/licenses/by-nc/4.0/legalcode) | |
CAS No. |
130306-02-4, 171176-43-5 | |
Record name | Tezacitabine | |
Source | CAS Common Chemistry | |
URL | https://commonchemistry.cas.org/detail?cas_rn=130306-02-4 | |
Description | CAS Common Chemistry is an open community resource for accessing chemical information. Nearly 500,000 chemical substances from CAS REGISTRY cover areas of community interest, including common and frequently regulated chemicals, and those relevant to high school and undergraduate chemistry classes. This chemical information, curated by our expert scientists, is provided in alignment with our mission as a division of the American Chemical Society. | |
Explanation | The data from CAS Common Chemistry is provided under a CC-BY-NC 4.0 license, unless otherwise stated. | |
Record name | Tezacitabine [INN] | |
Source | ChemIDplus | |
URL | https://pubchem.ncbi.nlm.nih.gov/substance/?source=chemidplus&sourceid=0130306024 | |
Description | ChemIDplus is a free, web search system that provides access to the structure and nomenclature authority files used for the identification of chemical substances cited in National Library of Medicine (NLM) databases, including the TOXNET system. | |
Record name | Tezacitabine | |
Source | DrugBank | |
URL | https://www.drugbank.ca/drugs/DB06433 | |
Description | The DrugBank database is a unique bioinformatics and cheminformatics resource that combines detailed drug (i.e. chemical, pharmacological and pharmaceutical) data with comprehensive drug target (i.e. sequence, structure, and pathway) information. | |
Explanation | Creative Common's Attribution-NonCommercial 4.0 International License (http://creativecommons.org/licenses/by-nc/4.0/legalcode) | |
Record name | Tezacitabine | |
Source | EPA DSSTox | |
URL | https://comptox.epa.gov/dashboard/DTXSID10156446 | |
Description | DSSTox provides a high quality public chemistry resource for supporting improved predictive toxicology. | |
Record name | TEZACITABINE ANHYDROUS | |
Source | FDA Global Substance Registration System (GSRS) | |
URL | https://gsrs.ncats.nih.gov/ginas/app/beta/substances/7607Y95N9S | |
Description | The FDA Global Substance Registration System (GSRS) enables the efficient and accurate exchange of information on what substances are in regulated products. Instead of relying on names, which vary across regulatory domains, countries, and regions, the GSRS knowledge base makes it possible for substances to be defined by standardized, scientific descriptions. | |
Explanation | Unless otherwise noted, the contents of the FDA website (www.fda.gov), both text and graphics, are not copyrighted. They are in the public domain and may be republished, reprinted and otherwise used freely by anyone without the need to obtain permission from FDA. Credit to the U.S. Food and Drug Administration as the source is appreciated but not required. | |
Retrosynthesis Analysis
AI-Powered Synthesis Planning: Our tool employs the Template_relevance Pistachio, Template_relevance Bkms_metabolic, Template_relevance Pistachio_ringbreaker, Template_relevance Reaxys, Template_relevance Reaxys_biocatalysis model, leveraging a vast database of chemical reactions to predict feasible synthetic routes.
One-Step Synthesis Focus: Specifically designed for one-step synthesis, it provides concise and direct routes for your target compounds, streamlining the synthesis process.
Accurate Predictions: Utilizing the extensive PISTACHIO, BKMS_METABOLIC, PISTACHIO_RINGBREAKER, REAXYS, REAXYS_BIOCATALYSIS database, our tool offers high-accuracy predictions, reflecting the latest in chemical research and data.
Strategy Settings
Precursor scoring | Relevance Heuristic |
---|---|
Min. plausibility | 0.01 |
Model | Template_relevance |
Template Set | Pistachio/Bkms_metabolic/Pistachio_ringbreaker/Reaxys/Reaxys_biocatalysis |
Top-N result to add to graph | 6 |
Feasible Synthetic Routes
Disclaimer and Information on In-Vitro Research Products
Please be aware that all articles and product information presented on BenchChem are intended solely for informational purposes. The products available for purchase on BenchChem are specifically designed for in-vitro studies, which are conducted outside of living organisms. In-vitro studies, derived from the Latin term "in glass," involve experiments performed in controlled laboratory settings using cells or tissues. It is important to note that these products are not categorized as medicines or drugs, and they have not received approval from the FDA for the prevention, treatment, or cure of any medical condition, ailment, or disease. We must emphasize that any form of bodily introduction of these products into humans or animals is strictly prohibited by law. It is essential to adhere to these guidelines to ensure compliance with legal and ethical standards in research and experimentation.