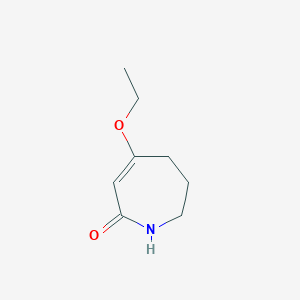
4-ethoxy-6,7-dihydro-1H-azepin-2(5H)-one
- Click on QUICK INQUIRY to receive a quote from our team of experts.
- With the quality product at a COMPETITIVE price, you can focus more on your research.
Overview
Description
4-ethoxy-6,7-dihydro-1H-azepin-2(5H)-one is a chemical compound that belongs to the class of heterocyclic compounds. It is also known as 4-ethoxy-5,6,7,8-tetrahydroazepin-2-one and is commonly used in scientific research.
Mechanism of Action
The exact mechanism of action of 4-ethoxy-6,7-dihydro-1H-azepin-2(5H)-one is not fully understood. However, it is believed to act on the central nervous system by modulating the activity of neurotransmitters such as serotonin, dopamine, and gamma-aminobutyric acid (GABA). It may also interact with ion channels and receptors in the brain.
Biochemical and Physiological Effects:
Studies have shown that 4-ethoxy-6,7-dihydro-1H-azepin-2(5H)-one has a range of biochemical and physiological effects. It has been shown to reduce inflammation and pain in animal models. It has also been found to have anticonvulsant activity and can reduce anxiety-like behaviors in rodents. Additionally, it has been shown to improve cognitive function in animal models of Alzheimer's disease.
Advantages and Limitations for Lab Experiments
One of the advantages of using 4-ethoxy-6,7-dihydro-1H-azepin-2(5H)-one in lab experiments is its relatively low toxicity. It is also relatively easy to synthesize and purify. However, one of the limitations is that it has not yet been extensively studied in humans, and its long-term safety and efficacy are not yet fully understood.
Future Directions
There are several future directions for research on 4-ethoxy-6,7-dihydro-1H-azepin-2(5H)-one. One area of interest is its potential use in the treatment of neurological disorders such as epilepsy, anxiety, and depression. Additionally, further studies are needed to fully understand its mechanism of action and to identify any potential side effects or drug interactions. Finally, there is a need for more studies to investigate its potential use as a therapeutic agent in humans.
Conclusion:
In conclusion, 4-ethoxy-6,7-dihydro-1H-azepin-2(5H)-one is a chemical compound that has been widely studied for its potential therapeutic applications. It has been shown to have anti-inflammatory, analgesic, and anticonvulsant properties and may have potential use in the treatment of neurological disorders. Further research is needed to fully understand its mechanism of action and to identify any potential side effects or drug interactions.
Synthesis Methods
The synthesis of 4-ethoxy-6,7-dihydro-1H-azepin-2(5H)-one involves the reaction of 5-ethyl-2,3-dihydro-1H-pyrrolin-2-one with ethyl bromoacetate. The reaction is carried out in the presence of a base such as potassium carbonate in anhydrous acetonitrile. The product is obtained after purification through column chromatography.
Scientific Research Applications
4-ethoxy-6,7-dihydro-1H-azepin-2(5H)-one has been widely used in scientific research for its potential therapeutic applications. It has been studied for its anti-inflammatory, analgesic, and anticonvulsant properties. It has also been investigated for its potential use in the treatment of anxiety, depression, and other neurological disorders.
properties
CAS RN |
165257-04-5 |
---|---|
Molecular Formula |
C8H13NO2 |
Molecular Weight |
155.19 g/mol |
IUPAC Name |
5-ethoxy-1,2,3,4-tetrahydroazepin-7-one |
InChI |
InChI=1S/C8H13NO2/c1-2-11-7-4-3-5-9-8(10)6-7/h6H,2-5H2,1H3,(H,9,10) |
InChI Key |
ZHQNCVSUZIZVDB-UHFFFAOYSA-N |
SMILES |
CCOC1=CC(=O)NCCC1 |
Canonical SMILES |
CCOC1=CC(=O)NCCC1 |
Origin of Product |
United States |
Disclaimer and Information on In-Vitro Research Products
Please be aware that all articles and product information presented on BenchChem are intended solely for informational purposes. The products available for purchase on BenchChem are specifically designed for in-vitro studies, which are conducted outside of living organisms. In-vitro studies, derived from the Latin term "in glass," involve experiments performed in controlled laboratory settings using cells or tissues. It is important to note that these products are not categorized as medicines or drugs, and they have not received approval from the FDA for the prevention, treatment, or cure of any medical condition, ailment, or disease. We must emphasize that any form of bodily introduction of these products into humans or animals is strictly prohibited by law. It is essential to adhere to these guidelines to ensure compliance with legal and ethical standards in research and experimentation.