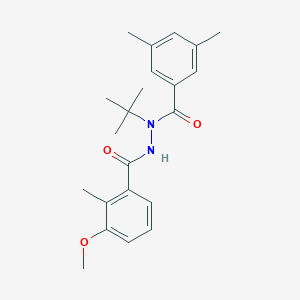
Methoxyfenozide
Overview
Description
Methoxyfenozide is a broad-spectrum insecticide . It is a substituted dibenzoylhydrazine that functions by accelerating the moulting process . It acts as an ecdysone agonist or ecdysonoid, substituting for the natural insect moulting hormone, 20-hydroxyecdysone .
Molecular Structure Analysis
This compound has a molecular formula of C22H28N2O3 . Its structure includes a diacylhydrazine moiety . The average mass is 368.469 Da and the monoisotopic mass is 368.209991 Da .
Physical And Chemical Properties Analysis
This compound is a solid, white powder . It has a melting point of 203.8 – 206.4 °C and decomposes at temperatures greater than 240 °C . It has a low solubility in water (3.3 ppm) but is soluble in various organic solvents .
Scientific Research Applications
Impact on Honey Bee Colony Activity and Thermoregulation
Methoxyfenozide, an insect growth regulator used in agriculture, has been studied for its effects on honey bees. Research indicates that exposure to this compound can disrupt honey bee colony flight activity and thermoregulation. This was observed in colonies exposed to this compound over a period of 9 weeks. The study highlights potential risks to bee colonies in agricultural settings where this pesticide is used (Meikle et al., 2019).
Biological and Chemical Properties
This compound functions as an ecdysteroid agonist in lepidopteran insects, mimicking the insect molting hormone. It shows high efficacy against various caterpillar pests and has a modest root systemic property. The chemical exhibits a high affinity to the ecdysone receptor complex in lepidopteran insects. Studies reveal its safety margin to non-target organisms, including beneficial insects (Carlson et al., 2001).
Resistance Risk in Musca Domestica
Research assessing resistance evolution in the house fly (Musca domestica) to this compound indicates a significant increase in resistance after continuous exposure. The study emphasizes the importance of managing the use of this compound to mitigate resistance development in pest populations (Shah et al., 2015).
Effects on Mosquito Reproduction
Studies on Culex pipiens, a medically important vector, show that this compound negatively impacts mosquito reproductive potential. Exposure to this chemical led to a reduction in fecundity and fertility, suggesting its utility in mosquito management with minimal ecotoxicological risks (Hamaidia & Soltani, 2020).
Biochemical Mechanisms of Resistance in Spodoptera Littoralis
A study on the cotton leafworm, Spodoptera littoralis, revealed that resistance to this compound is associated with increased monooxygenase activity. This finding is crucial for developing strategies against resistance in pests targeted by this insecticide (Mosallanejad & Smagghe, 2009).
Mechanism of Action
Safety and Hazards
Methoxyfenozide is classified as hazardous to the aquatic environment . It is very toxic to aquatic life and can have long-lasting effects . Therefore, it’s important to avoid release to the environment . In case of accidental release, it’s recommended to collect spillage and dispose of it in accordance with local regulations .
Future Directions
Methoxyfenozide is being studied for its potential in nanoscale delivery systems for more effective pest control . Such systems could improve absorption, coverage, and permeability while protecting the insecticide from abiotic conditions and extending its efficacy through controlled release . This could lead to more sustainable and effective pest control strategies in the future .
properties
IUPAC Name |
N'-tert-butyl-N'-(3,5-dimethylbenzoyl)-3-methoxy-2-methylbenzohydrazide | |
---|---|---|
Source | PubChem | |
URL | https://pubchem.ncbi.nlm.nih.gov | |
Description | Data deposited in or computed by PubChem | |
InChI |
InChI=1S/C22H28N2O3/c1-14-11-15(2)13-17(12-14)21(26)24(22(4,5)6)23-20(25)18-9-8-10-19(27-7)16(18)3/h8-13H,1-7H3,(H,23,25) | |
Source | PubChem | |
URL | https://pubchem.ncbi.nlm.nih.gov | |
Description | Data deposited in or computed by PubChem | |
InChI Key |
QCAWEPFNJXQPAN-UHFFFAOYSA-N | |
Source | PubChem | |
URL | https://pubchem.ncbi.nlm.nih.gov | |
Description | Data deposited in or computed by PubChem | |
Canonical SMILES |
CC1=CC(=CC(=C1)C(=O)N(C(C)(C)C)NC(=O)C2=C(C(=CC=C2)OC)C)C | |
Source | PubChem | |
URL | https://pubchem.ncbi.nlm.nih.gov | |
Description | Data deposited in or computed by PubChem | |
Molecular Formula |
C22H28N2O3 | |
Source | PubChem | |
URL | https://pubchem.ncbi.nlm.nih.gov | |
Description | Data deposited in or computed by PubChem | |
DSSTOX Substance ID |
DTXSID3032628 | |
Record name | Methoxyfenozide | |
Source | EPA DSSTox | |
URL | https://comptox.epa.gov/dashboard/DTXSID3032628 | |
Description | DSSTox provides a high quality public chemistry resource for supporting improved predictive toxicology. | |
Molecular Weight |
368.5 g/mol | |
Source | PubChem | |
URL | https://pubchem.ncbi.nlm.nih.gov | |
Description | Data deposited in or computed by PubChem | |
Solubility |
In DMSO 11, cyclohexanone 9.9, acetone 9 (all in g/100g at 20 °C), In water, 3.3 mg/L at 20 °C | |
Record name | Methoxyfenozide | |
Source | Hazardous Substances Data Bank (HSDB) | |
URL | https://pubchem.ncbi.nlm.nih.gov/source/hsdb/7936 | |
Description | The Hazardous Substances Data Bank (HSDB) is a toxicology database that focuses on the toxicology of potentially hazardous chemicals. It provides information on human exposure, industrial hygiene, emergency handling procedures, environmental fate, regulatory requirements, nanomaterials, and related areas. The information in HSDB has been assessed by a Scientific Review Panel. | |
Color/Form |
White powder | |
CAS RN |
161050-58-4 | |
Record name | Methoxyfenozide | |
Source | CAS Common Chemistry | |
URL | https://commonchemistry.cas.org/detail?cas_rn=161050-58-4 | |
Description | CAS Common Chemistry is an open community resource for accessing chemical information. Nearly 500,000 chemical substances from CAS REGISTRY cover areas of community interest, including common and frequently regulated chemicals, and those relevant to high school and undergraduate chemistry classes. This chemical information, curated by our expert scientists, is provided in alignment with our mission as a division of the American Chemical Society. | |
Explanation | The data from CAS Common Chemistry is provided under a CC-BY-NC 4.0 license, unless otherwise stated. | |
Record name | Methoxyfenozide [ISO:ANSI:BSI] | |
Source | ChemIDplus | |
URL | https://pubchem.ncbi.nlm.nih.gov/substance/?source=chemidplus&sourceid=0161050584 | |
Description | ChemIDplus is a free, web search system that provides access to the structure and nomenclature authority files used for the identification of chemical substances cited in National Library of Medicine (NLM) databases, including the TOXNET system. | |
Record name | Methoxyfenozide | |
Source | EPA DSSTox | |
URL | https://comptox.epa.gov/dashboard/DTXSID3032628 | |
Description | DSSTox provides a high quality public chemistry resource for supporting improved predictive toxicology. | |
Record name | Benzoic acid, 3-methoxy-2-methyl-, 2-(3,5-dimethylbenzoyl)-2-(1,1-dimethylethyl)hydrazide | |
Source | European Chemicals Agency (ECHA) | |
URL | https://echa.europa.eu/substance-information/-/substanceinfo/100.120.252 | |
Description | The European Chemicals Agency (ECHA) is an agency of the European Union which is the driving force among regulatory authorities in implementing the EU's groundbreaking chemicals legislation for the benefit of human health and the environment as well as for innovation and competitiveness. | |
Explanation | Use of the information, documents and data from the ECHA website is subject to the terms and conditions of this Legal Notice, and subject to other binding limitations provided for under applicable law, the information, documents and data made available on the ECHA website may be reproduced, distributed and/or used, totally or in part, for non-commercial purposes provided that ECHA is acknowledged as the source: "Source: European Chemicals Agency, http://echa.europa.eu/". Such acknowledgement must be included in each copy of the material. ECHA permits and encourages organisations and individuals to create links to the ECHA website under the following cumulative conditions: Links can only be made to webpages that provide a link to the Legal Notice page. | |
Record name | METHOXYFENOZIDE | |
Source | FDA Global Substance Registration System (GSRS) | |
URL | https://gsrs.ncats.nih.gov/ginas/app/beta/substances/62A22651ZX | |
Description | The FDA Global Substance Registration System (GSRS) enables the efficient and accurate exchange of information on what substances are in regulated products. Instead of relying on names, which vary across regulatory domains, countries, and regions, the GSRS knowledge base makes it possible for substances to be defined by standardized, scientific descriptions. | |
Explanation | Unless otherwise noted, the contents of the FDA website (www.fda.gov), both text and graphics, are not copyrighted. They are in the public domain and may be republished, reprinted and otherwise used freely by anyone without the need to obtain permission from FDA. Credit to the U.S. Food and Drug Administration as the source is appreciated but not required. | |
Record name | Methoxyfenozide | |
Source | Hazardous Substances Data Bank (HSDB) | |
URL | https://pubchem.ncbi.nlm.nih.gov/source/hsdb/7936 | |
Description | The Hazardous Substances Data Bank (HSDB) is a toxicology database that focuses on the toxicology of potentially hazardous chemicals. It provides information on human exposure, industrial hygiene, emergency handling procedures, environmental fate, regulatory requirements, nanomaterials, and related areas. The information in HSDB has been assessed by a Scientific Review Panel. | |
Melting Point |
206.2 - 208 °C, MP: 204 - 20.6 °C /Technical/ | |
Record name | Methoxyfenozide | |
Source | Hazardous Substances Data Bank (HSDB) | |
URL | https://pubchem.ncbi.nlm.nih.gov/source/hsdb/7936 | |
Description | The Hazardous Substances Data Bank (HSDB) is a toxicology database that focuses on the toxicology of potentially hazardous chemicals. It provides information on human exposure, industrial hygiene, emergency handling procedures, environmental fate, regulatory requirements, nanomaterials, and related areas. The information in HSDB has been assessed by a Scientific Review Panel. | |
Synthesis routes and methods I
Procedure details
Synthesis routes and methods II
Procedure details
Retrosynthesis Analysis
AI-Powered Synthesis Planning: Our tool employs the Template_relevance Pistachio, Template_relevance Bkms_metabolic, Template_relevance Pistachio_ringbreaker, Template_relevance Reaxys, Template_relevance Reaxys_biocatalysis model, leveraging a vast database of chemical reactions to predict feasible synthetic routes.
One-Step Synthesis Focus: Specifically designed for one-step synthesis, it provides concise and direct routes for your target compounds, streamlining the synthesis process.
Accurate Predictions: Utilizing the extensive PISTACHIO, BKMS_METABOLIC, PISTACHIO_RINGBREAKER, REAXYS, REAXYS_BIOCATALYSIS database, our tool offers high-accuracy predictions, reflecting the latest in chemical research and data.
Strategy Settings
Precursor scoring | Relevance Heuristic |
---|---|
Min. plausibility | 0.01 |
Model | Template_relevance |
Template Set | Pistachio/Bkms_metabolic/Pistachio_ringbreaker/Reaxys/Reaxys_biocatalysis |
Top-N result to add to graph | 6 |
Feasible Synthetic Routes
Disclaimer and Information on In-Vitro Research Products
Please be aware that all articles and product information presented on BenchChem are intended solely for informational purposes. The products available for purchase on BenchChem are specifically designed for in-vitro studies, which are conducted outside of living organisms. In-vitro studies, derived from the Latin term "in glass," involve experiments performed in controlled laboratory settings using cells or tissues. It is important to note that these products are not categorized as medicines or drugs, and they have not received approval from the FDA for the prevention, treatment, or cure of any medical condition, ailment, or disease. We must emphasize that any form of bodily introduction of these products into humans or animals is strictly prohibited by law. It is essential to adhere to these guidelines to ensure compliance with legal and ethical standards in research and experimentation.