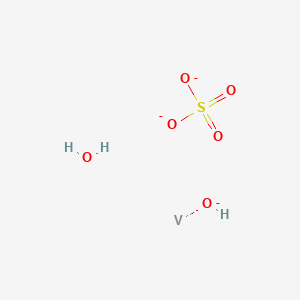
Oxidovanadium(3+) sulfate hydrate
Overview
Description
Oxidovanadium(3+) sulfate hydrate is a chemical compound that has garnered significant attention in various scientific fields due to its unique properties and potential applications. This compound consists of vanadium in the +3 oxidation state, coordinated with sulfate ions and water molecules. It is known for its vibrant color and its role in various chemical reactions and industrial processes.
Mechanism of Action
Target of Action
Oxidovanadium(3+) sulfate hydrate, like other vanadium compounds, has been shown to have a variety of biological effects . The primary targets of this compound are often proteins and enzymes in the body. For instance, it has been found to interact with insulin receptors, enhancing their activity . This interaction plays a crucial role in the compound’s antidiabetic properties .
Mode of Action
The mode of action of this compound involves its interaction with its targets, leading to changes in their function. For instance, when interacting with insulin receptors, it enhances their activity, leading to increased insulin sensitivity . This results in improved glucose uptake and metabolism, which is beneficial for individuals with diabetes .
Biochemical Pathways
The compound affects several biochemical pathways. One of the key pathways is the insulin signaling pathway. By enhancing the activity of insulin receptors, this compound improves the efficiency of this pathway, leading to better glucose metabolism . Additionally, it has been found to be involved in the CO2 cycloaddition reaction with epoxides, resulting in up to 100% cyclic carbonate products .
Pharmacokinetics
It is known that vanadium compounds generally have poor absorption in the gastrointestinal tract
Result of Action
The result of the action of this compound is primarily seen in its antidiabetic effects. It enhances insulin sensitivity, leading to improved glucose metabolism . This can help to regulate blood glucose levels in individuals with diabetes. Additionally, it has been found to catalyze the CO2 cycloaddition reaction with epoxides, leading to the formation of cyclic carbonate products .
Action Environment
The action of this compound can be influenced by various environmental factors. For instance, its antidiabetic effects are more pronounced under hyperglycemic conditions . Additionally, the efficiency of its catalytic activity in the CO2 cycloaddition reaction may be influenced by factors such as temperature and pH
Biochemical Analysis
Biochemical Properties
Oxidovanadium(3+) sulfate hydrate has been found to interact with various enzymes, proteins, and other biomolecules . For instance, it has been shown to play a role in lipid, DNA, and protein synthesis, glucose transport and metabolism, and insulin-mimetic activity . It also has mitogenic effects on different types of cells .
Cellular Effects
The effects of this compound on cells are diverse and significant . It has been found to have antitumor properties, inhibiting the viability of osteosarcoma cells in a dose-dependent manner . It also influences cell function by affecting cell signaling pathways, gene expression, and cellular metabolism .
Molecular Mechanism
At the molecular level, this compound exerts its effects through various mechanisms . It has been found to bind with biomolecules, inhibit or activate enzymes, and cause changes in gene expression . For example, it has been identified as an efficient catalyst for the CO2 cycloaddition reaction with epoxides .
Temporal Effects in Laboratory Settings
In laboratory settings, the effects of this compound can change over time . It has been observed that the compound’s stability, degradation, and long-term effects on cellular function can vary in in vitro or in vivo studies .
Dosage Effects in Animal Models
The effects of this compound can vary with different dosages in animal models . For instance, it has been found that the compound can improve glycemia in streptozotocin-induced diabetic rats when administered at a dosage of 30 mg/kg .
Metabolic Pathways
This compound is involved in various metabolic pathways . It interacts with enzymes or cofactors and can affect metabolic flux or metabolite levels .
Transport and Distribution
This compound is transported and distributed within cells and tissues . It can interact with transporters or binding proteins, and can affect its localization or accumulation .
Subcellular Localization
The subcellular localization of this compound can affect its activity or function . It could include any targeting signals or post-translational modifications that direct it to specific compartments or organelles .
Preparation Methods
Synthetic Routes and Reaction Conditions
The synthesis of oxidovanadium(3+) sulfate hydrate typically involves the reaction of vanadium(III) oxide with sulfuric acid in the presence of water. The reaction is carried out under controlled conditions to ensure the formation of the desired hydrate. The general reaction can be represented as: [ \text{V}_2\text{O}_3 + 3\text{H}_2\text{SO}_4 + x\text{H}_2\text{O} \rightarrow 2\text{VSO}_4 \cdot x\text{H}_2\text{O} + 3\text{H}_2\text{O} ]
Industrial Production Methods
In industrial settings, the production of this compound involves large-scale reactions using high-purity vanadium sources and sulfuric acid. The process is optimized to maximize yield and purity, often involving multiple purification steps such as crystallization and filtration.
Chemical Reactions Analysis
Types of Reactions
Oxidovanadium(3+) sulfate hydrate undergoes various types of chemical reactions, including:
Oxidation: The compound can be oxidized to higher oxidation states of vanadium, such as vanadium(IV) or vanadium(V).
Reduction: It can be reduced to lower oxidation states, although this is less common.
Substitution: Ligand exchange reactions where the sulfate or water ligands are replaced by other ligands.
Common Reagents and Conditions
Common reagents used in reactions with this compound include oxidizing agents like hydrogen peroxide and reducing agents like sodium borohydride. The reactions are typically carried out in aqueous solutions under controlled temperatures and pH conditions.
Major Products Formed
The major products formed from these reactions depend on the specific reagents and conditions used. For example, oxidation reactions can yield vanadium(IV) oxide sulfate or vanadium(V) oxide, while substitution reactions can produce various vanadium complexes with different ligands.
Scientific Research Applications
Oxidovanadium(3+) sulfate hydrate has a wide range of applications in scientific research:
Comparison with Similar Compounds
Similar Compounds
Vanadium(IV) oxide sulfate hydrate: Similar in structure but with vanadium in the +4 oxidation state.
Vanadium(V) oxide: A higher oxidation state compound with different reactivity and applications.
Vanadium(III) chloride: Another vanadium(III) compound with chloride ligands instead of sulfate.
Uniqueness
Oxidovanadium(3+) sulfate hydrate is unique due to its specific oxidation state and coordination environment, which confer distinct chemical and biological properties. Its ability to undergo various redox reactions and form stable complexes with different ligands makes it a versatile compound in both research and industrial applications .
Biological Activity
Oxidovanadium(3+) sulfate hydrate is a compound of increasing interest in the field of medicinal chemistry due to its potential biological activities. Vanadium compounds, particularly oxidovanadium complexes, have been studied for their anticancer, anti-diabetic, and antimicrobial properties. This article aims to provide a comprehensive overview of the biological activity associated with this compound, including synthesis, characterization, and research findings.
Synthesis and Characterization
This compound can be synthesized through various methods, often involving the reaction of vanadium salts with sulfate ions in aqueous solutions. Characterization techniques such as infrared (IR) spectroscopy, nuclear magnetic resonance (NMR), and X-ray crystallography are utilized to confirm the structure and purity of the synthesized compound.
Table 1: Characterization Techniques for this compound
Technique | Purpose |
---|---|
IR Spectroscopy | Identify functional groups |
NMR | Determine molecular structure |
X-ray Crystallography | Confirm crystal structure |
Mass Spectrometry | Analyze molecular weight |
Anticancer Activity
Research indicates that oxidovanadium compounds exhibit significant anticancer properties. Studies have shown that these compounds can inhibit the growth of various cancer cell lines, including hepatocellular carcinoma (HepG-2) and breast adenocarcinoma (MCF-7). The mechanism often involves interference with cellular signaling pathways and induction of apoptosis.
- Case Study : A study reported an IC50 value for oxidovanadium(3+) complexes against HepG-2 cells at approximately 25 µg/mL, indicating potent cytotoxic effects .
Antimicrobial Activity
This compound has demonstrated antimicrobial activity against a range of pathogenic bacteria and fungi. The minimum inhibitory concentration (MIC) method is commonly employed to assess this activity.
- Findings : In vitro studies revealed that oxidovanadium complexes exhibited enhanced antimicrobial effects against bacteria such as E. coli, S. aureus, and fungi like C. albicans compared to free ligands .
Table 2: Antimicrobial Activity of Oxidovanadium(3+) Compounds
Pathogen | MIC (µg/mL) |
---|---|
E. coli | 15 |
S. aureus | 10 |
C. albicans | 20 |
Mechanistic Insights
The biological activity of oxidovanadium compounds is attributed to their ability to mimic insulin action and modulate various biochemical pathways. They can influence glucose metabolism, enhance insulin sensitivity, and exhibit antioxidant properties.
- Molecular Docking Studies : Theoretical investigations using density functional theory (DFT) have provided insights into the binding interactions between oxidovanadium complexes and target biomolecules, supporting their potential as therapeutic agents .
Toxicity Studies
While the biological activities are promising, toxicity assessments are crucial for evaluating the safety profile of oxidovanadium compounds. Studies indicate that certain complexes exhibit low toxicity levels in vivo, with LD50 values suggesting they fall within the category of slightly toxic substances .
Properties
CAS No. |
123334-20-3 |
---|---|
Molecular Formula |
H2O6SV |
Molecular Weight |
181.02 g/mol |
IUPAC Name |
oxovanadium(2+);sulfate;hydrate |
InChI |
InChI=1S/H2O4S.H2O.O.V/c1-5(2,3)4;;;/h(H2,1,2,3,4);1H2;;/q;;;+2/p-2 |
InChI Key |
DKCWBFMZNUOFEM-UHFFFAOYSA-L |
SMILES |
O.[OH-].[O-]S(=O)(=O)[O-].[V] |
Canonical SMILES |
O.[O-]S(=O)(=O)[O-].O=[V+2] |
Related CAS |
12439-96-2 16840-96-3 |
Origin of Product |
United States |
Retrosynthesis Analysis
AI-Powered Synthesis Planning: Our tool employs the Template_relevance Pistachio, Template_relevance Bkms_metabolic, Template_relevance Pistachio_ringbreaker, Template_relevance Reaxys, Template_relevance Reaxys_biocatalysis model, leveraging a vast database of chemical reactions to predict feasible synthetic routes.
One-Step Synthesis Focus: Specifically designed for one-step synthesis, it provides concise and direct routes for your target compounds, streamlining the synthesis process.
Accurate Predictions: Utilizing the extensive PISTACHIO, BKMS_METABOLIC, PISTACHIO_RINGBREAKER, REAXYS, REAXYS_BIOCATALYSIS database, our tool offers high-accuracy predictions, reflecting the latest in chemical research and data.
Strategy Settings
Precursor scoring | Relevance Heuristic |
---|---|
Min. plausibility | 0.01 |
Model | Template_relevance |
Template Set | Pistachio/Bkms_metabolic/Pistachio_ringbreaker/Reaxys/Reaxys_biocatalysis |
Top-N result to add to graph | 6 |
Feasible Synthetic Routes
Disclaimer and Information on In-Vitro Research Products
Please be aware that all articles and product information presented on BenchChem are intended solely for informational purposes. The products available for purchase on BenchChem are specifically designed for in-vitro studies, which are conducted outside of living organisms. In-vitro studies, derived from the Latin term "in glass," involve experiments performed in controlled laboratory settings using cells or tissues. It is important to note that these products are not categorized as medicines or drugs, and they have not received approval from the FDA for the prevention, treatment, or cure of any medical condition, ailment, or disease. We must emphasize that any form of bodily introduction of these products into humans or animals is strictly prohibited by law. It is essential to adhere to these guidelines to ensure compliance with legal and ethical standards in research and experimentation.