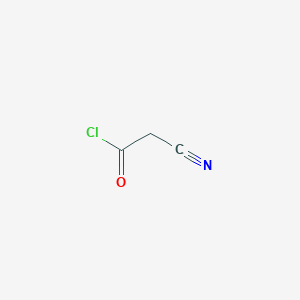
2-cyanoacetyl Chloride
Overview
Description
2-Cyanoacetyl chloride is an organic compound with the molecular formula C3H2ClNO. It is a reactive chemical used in various organic synthesis processes. The compound is characterized by the presence of a cyano group (C≡N) and an acyl chloride group (C=O)Cl, making it highly reactive and useful in the formation of other chemical compounds.
Mechanism of Action
Target of Action
2-Cyanoacetyl Chloride is primarily used as a reactant in the synthesis of various heterocyclic compounds . It is particularly effective in the cyanoacetylation of amines, which is a key step in the formation of biologically active compounds .
Mode of Action
The compound interacts with its targets (amines) through a process known as cyanoacetylation . This involves the carbonyl and cyano functions of this compound reacting with common bidentate reagents to form a variety of heterocyclic compounds .
Biochemical Pathways
The active hydrogen on C-2 of these compounds can take part in a variety of condensation and substitution reactions . This leads to the formation of various organic heterocycles, which are key components in many biochemical pathways .
Result of Action
The result of this compound’s action is the formation of a variety of heterocyclic compounds . These compounds are often biologically active and can serve as precursors for the synthesis of various chemotherapeutic agents .
Action Environment
The action of this compound is influenced by various environmental factors, including the presence of other reactants, temperature, and reaction conditions . For example, the compound has been used in reactions carried out at room temperature, as well as in reactions involving heat .
Preparation Methods
Synthetic Routes and Reaction Conditions: 2-Cyanoacetyl chloride can be synthesized through the reaction of cyanoacetic acid with thionyl chloride. The reaction typically occurs under reflux conditions, where thionyl chloride acts as both a chlorinating agent and a dehydrating agent. The general reaction is as follows:
NC-CH2COOH+SOCl2→NC-CH2COCl+SO2+HCl
This reaction is carried out under anhydrous conditions to prevent the hydrolysis of the product.
Industrial Production Methods: In industrial settings, the production of this compound involves similar reaction conditions but on a larger scale. The use of continuous flow reactors can enhance the efficiency and yield of the product. Additionally, the reaction is carefully controlled to manage the release of by-products such as sulfur dioxide and hydrogen chloride.
Chemical Reactions Analysis
Types of Reactions: 2-Cyanoacetyl chloride undergoes various chemical reactions, including:
Acylation: It reacts with alcohols, phenols, and amines to form esters, amides, and imides, respectively.
Hydrolysis: In the presence of water, it hydrolyzes to form cyanoacetic acid and hydrochloric acid.
Condensation Reactions: It can participate in condensation reactions with compounds containing active hydrogen atoms, such as amines and hydrazines, to form cyanoacetamides and cyanoacetohydrazides.
Common Reagents and Conditions:
Acylation: Typically carried out in the presence of a base such as triethylamine to neutralize the hydrochloric acid formed during the reaction.
Hydrolysis: Conducted under aqueous conditions, often at elevated temperatures to accelerate the reaction.
Condensation: Requires the presence of a catalyst or a base to facilitate the reaction.
Major Products:
Esters and Amides: Formed through acylation reactions.
Cyanoacetic Acid: Produced via hydrolysis.
Cyanoacetamides and Cyanoacetohydrazides: Result from condensation reactions.
Scientific Research Applications
2-Cyanoacetyl chloride is utilized in various scientific research applications, including:
Organic Synthesis: It serves as a building block for the synthesis of more complex organic molecules, including heterocyclic compounds.
Pharmaceutical Research: Used in the synthesis of potential drug candidates, particularly those involving cyanoacetamide derivatives.
Material Science: Employed in the preparation of polymers and other advanced materials.
Biochemistry: Investigated for its role in metabolic pathways and as a potential activator of certain enzymes.
Comparison with Similar Compounds
Cyanoacetic Acid: Similar in structure but lacks the acyl chloride group, making it less reactive.
Cyanoacetamide: Contains an amide group instead of an acyl chloride, leading to different reactivity patterns.
Cyanoacetohydrazide: Features a hydrazide group, which alters its chemical behavior compared to 2-cyanoacetyl chloride.
Uniqueness: this compound is unique due to its dual functional groups (cyano and acyl chloride), which provide a versatile platform for various chemical transformations. Its high reactivity makes it a valuable intermediate in organic synthesis, particularly for the formation of cyanoacetamide derivatives and other heterocyclic compounds.
Biological Activity
2-Cyanoacetyl chloride (C₃H₂ClNO) is an organic compound characterized by its cyano and acyl chloride functional groups. Its high reactivity makes it a valuable intermediate in organic synthesis, particularly in the pharmaceutical and material sciences. This article explores the biological activity of this compound, focusing on its mechanisms of action, applications in drug development, and relevant research findings.
- Molecular Formula : C₃H₂ClNO
- Molecular Weight : 103.5 g/mol
- Appearance : Colorless to yellow liquid
- Boiling Point : 143 °C
- Reactivity : Highly reactive due to the presence of both cyano and acyl chloride groups, facilitating various condensation and substitution reactions.
This compound primarily acts as a cyanoacetylating agent. It interacts with nucleophiles, such as amines, through a process known as cyanoacetylation , leading to the formation of various heterocyclic compounds. The active hydrogen on C-2 of these compounds can participate in multiple biochemical pathways, influencing metabolic processes and enzyme activation.
Pharmaceutical Research
This compound is utilized in synthesizing potential drug candidates, particularly derivatives of cyanoacetamide. Research indicates that compounds derived from this compound exhibit significant biological activities, including:
- Antioxidant Activity : Compounds synthesized from this compound have demonstrated antioxidant properties. For instance, derivatives showed up to 50.3% scavenging activity against DPPH radicals, comparable to standard antioxidants like ascorbic acid .
- Antibacterial Activity : In vitro studies have shown that synthesized compounds possess moderate to good antibacterial activity against both Gram-negative (E. coli) and Gram-positive (S. aureus) bacteria .
Case Studies
- Cyanoacetylation of Substituted 2-Aminothiophenes :
- Utilization in Heterocyclic Compound Synthesis :
Table: Biological Activities of Compounds Derived from this compound
Compound | Antioxidant Activity (%) | Antibacterial Activity (E. coli) | Antibacterial Activity (S. aureus) |
---|---|---|---|
2-(2-cyanoacetamido)-4,5-dimethylthiophene-3-carboxamide | 50.3 | Moderate | Good |
Other derivatives | Varies | Moderate to Good | Moderate to Good |
Properties
IUPAC Name |
2-cyanoacetyl chloride | |
---|---|---|
Source | PubChem | |
URL | https://pubchem.ncbi.nlm.nih.gov | |
Description | Data deposited in or computed by PubChem | |
InChI |
InChI=1S/C3H2ClNO/c4-3(6)1-2-5/h1H2 | |
Source | PubChem | |
URL | https://pubchem.ncbi.nlm.nih.gov | |
Description | Data deposited in or computed by PubChem | |
InChI Key |
GEQZTCMVWVDEDF-UHFFFAOYSA-N | |
Source | PubChem | |
URL | https://pubchem.ncbi.nlm.nih.gov | |
Description | Data deposited in or computed by PubChem | |
Canonical SMILES |
C(C#N)C(=O)Cl | |
Source | PubChem | |
URL | https://pubchem.ncbi.nlm.nih.gov | |
Description | Data deposited in or computed by PubChem | |
Molecular Formula |
C3H2ClNO | |
Source | PubChem | |
URL | https://pubchem.ncbi.nlm.nih.gov | |
Description | Data deposited in or computed by PubChem | |
Molecular Weight |
103.51 g/mol | |
Source | PubChem | |
URL | https://pubchem.ncbi.nlm.nih.gov | |
Description | Data deposited in or computed by PubChem | |
CAS No. |
16130-58-8 | |
Record name | 2-cyanoacetyl chloride | |
Source | European Chemicals Agency (ECHA) | |
URL | https://echa.europa.eu/information-on-chemicals | |
Description | The European Chemicals Agency (ECHA) is an agency of the European Union which is the driving force among regulatory authorities in implementing the EU's groundbreaking chemicals legislation for the benefit of human health and the environment as well as for innovation and competitiveness. | |
Explanation | Use of the information, documents and data from the ECHA website is subject to the terms and conditions of this Legal Notice, and subject to other binding limitations provided for under applicable law, the information, documents and data made available on the ECHA website may be reproduced, distributed and/or used, totally or in part, for non-commercial purposes provided that ECHA is acknowledged as the source: "Source: European Chemicals Agency, http://echa.europa.eu/". Such acknowledgement must be included in each copy of the material. ECHA permits and encourages organisations and individuals to create links to the ECHA website under the following cumulative conditions: Links can only be made to webpages that provide a link to the Legal Notice page. | |
Retrosynthesis Analysis
AI-Powered Synthesis Planning: Our tool employs the Template_relevance Pistachio, Template_relevance Bkms_metabolic, Template_relevance Pistachio_ringbreaker, Template_relevance Reaxys, Template_relevance Reaxys_biocatalysis model, leveraging a vast database of chemical reactions to predict feasible synthetic routes.
One-Step Synthesis Focus: Specifically designed for one-step synthesis, it provides concise and direct routes for your target compounds, streamlining the synthesis process.
Accurate Predictions: Utilizing the extensive PISTACHIO, BKMS_METABOLIC, PISTACHIO_RINGBREAKER, REAXYS, REAXYS_BIOCATALYSIS database, our tool offers high-accuracy predictions, reflecting the latest in chemical research and data.
Strategy Settings
Precursor scoring | Relevance Heuristic |
---|---|
Min. plausibility | 0.01 |
Model | Template_relevance |
Template Set | Pistachio/Bkms_metabolic/Pistachio_ringbreaker/Reaxys/Reaxys_biocatalysis |
Top-N result to add to graph | 6 |
Feasible Synthetic Routes
Disclaimer and Information on In-Vitro Research Products
Please be aware that all articles and product information presented on BenchChem are intended solely for informational purposes. The products available for purchase on BenchChem are specifically designed for in-vitro studies, which are conducted outside of living organisms. In-vitro studies, derived from the Latin term "in glass," involve experiments performed in controlled laboratory settings using cells or tissues. It is important to note that these products are not categorized as medicines or drugs, and they have not received approval from the FDA for the prevention, treatment, or cure of any medical condition, ailment, or disease. We must emphasize that any form of bodily introduction of these products into humans or animals is strictly prohibited by law. It is essential to adhere to these guidelines to ensure compliance with legal and ethical standards in research and experimentation.