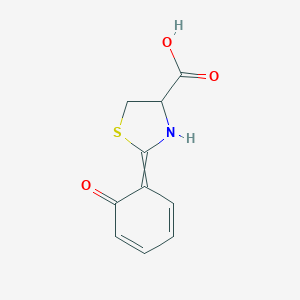
Dihydroaeruginoic acid
Overview
Description
Etoposide quinone is a metabolite of the anticancer drug etoposide. Etoposide is a semisynthetic derivative of podophyllotoxin, which is used to treat various types of cancer, including testicular cancer, small cell lung cancer, and other malignancies. Etoposide quinone is known for its role in the development of secondary acute myeloid leukemias in some patients treated with etoposide .
Preparation Methods
Synthetic Routes and Reaction Conditions: Etoposide quinone is synthesized from etoposide through metabolic oxidation. The process involves the conversion of etoposide to etoposide catechol by the enzyme cytochrome P450 3A4 (CYP3A4), followed by further oxidation to etoposide quinone .
Industrial Production Methods: The industrial production of etoposide quinone involves the large-scale synthesis of etoposide, followed by its metabolic conversion to etoposide quinone. The process requires stringent control of reaction conditions to ensure the purity and yield of the final product .
Chemical Reactions Analysis
Types of Reactions: Etoposide quinone undergoes various chemical reactions, including oxidation, reduction, and substitution. It is known to form covalent bonds with proteins and DNA, leading to its biological activity .
Common Reagents and Conditions:
Oxidation: Etoposide is oxidized to etoposide quinone using enzymes such as CYP3A4.
Reduction: Reducing agents can reverse the oxidation process, converting etoposide quinone back to etoposide catechol.
Substitution: Etoposide quinone can react with nucleophiles, leading to the formation of covalent adducts.
Major Products: The major product of the oxidation of etoposide is etoposide quinone. The reduction of etoposide quinone yields etoposide catechol .
Scientific Research Applications
Etoposide quinone has several scientific research applications:
Chemistry: It is used to study the mechanisms of enzyme-mediated oxidation and reduction reactions.
Biology: Etoposide quinone is used to investigate the role of topoisomerase II in DNA cleavage and repair.
Medicine: It is studied for its role in the development of secondary acute myeloid leukemias and its potential as a chemotherapeutic agent.
Mechanism of Action
Etoposide quinone acts as a covalent poison of human topoisomerase IIβ. It induces DNA cleavage by forming covalent bonds with the enzyme, leading to the accumulation of double-stranded breaks in the DNA. This process ultimately results in cell death. Etoposide quinone is also a potent irreversible inhibitor of TCPTP protein tyrosine phosphatase, contributing to its leukemogenic effects .
Comparison with Similar Compounds
Etoposide quinone is unique in its ability to form covalent bonds with topoisomerase IIβ, leading to a higher ratio of double- to single-stranded DNA breaks compared to its parent compound, etoposide. Similar compounds include:
Doxorubicin: Another topoisomerase II inhibitor used in cancer treatment.
Mitoxantrone: A topoisomerase II inhibitor with a different mechanism of action.
Podophyllotoxin: The natural precursor of etoposide, used in the synthesis of various anticancer drugs.
Etoposide quinone stands out due to its specific interaction with topoisomerase IIβ and its role in the development of secondary leukemias.
Biological Activity
Dihydroaeruginoic acid (Dha) is a bioactive compound primarily produced by certain strains of Pseudomonas fluorescens. It has garnered attention due to its significant biological activities, particularly its antimicrobial properties and its role in iron acquisition as a siderophore. This article explores the biological activity of this compound, supported by various research findings, case studies, and data tables.
Chemical Structure and Synthesis
This compound is synthesized from salicylate and cysteine through the action of specific synthetases encoded by the pchEF genes in Pseudomonas aeruginosa. The enzyme this compound synthetase (PchE) catalyzes the conversion of salicylate to dihydroaeruginoate, which is then further processed to pyochelin, a well-known siderophore that aids in iron uptake under low-iron conditions .
Antimicrobial Properties
This compound exhibits notable antimicrobial activity against various phytopathogenic fungi and bacteria. Research has demonstrated its effectiveness as an inhibitor of Septoria tritici, a significant pathogen affecting wheat crops. In vitro studies have shown that Dha can inhibit the growth of this fungus as well as other pathogens such as Rhizoctonia solani .
Table 1: Antimicrobial Activity of this compound
Role in Iron Acquisition
As a siderophore, this compound plays a crucial role in iron acquisition for Pseudomonas fluorescens. It binds iron ions with high affinity, facilitating their uptake in environments where iron is limited. This function is vital for the survival and competitiveness of these bacteria in various ecological niches .
Case Studies
- Inhibition of Plant Pathogens : A study conducted by Cox et al. (1981) highlighted the effectiveness of this compound against Septoria tritici, demonstrating its potential use as a biocontrol agent in agriculture. The study reported significant reductions in fungal biomass when treated with Dha, suggesting its application in sustainable farming practices .
- Siderophore Activity : Research by Serino et al. (1995) focused on the siderophore-like activity of this compound. The findings indicated that Dha not only aids in iron uptake but also enhances the growth of beneficial microbes in the rhizosphere, promoting plant health .
The mechanisms through which this compound exerts its biological effects include:
- Inhibition of Enzyme Activity : Dha interferes with key metabolic pathways in pathogens, leading to reduced growth and viability.
- Iron Chelation : By binding to iron, Dha deprives pathogens of this essential nutrient, inhibiting their growth.
- Induction of Systemic Resistance : In plants, Dha may trigger systemic resistance mechanisms against various pathogens, enhancing overall plant defense .
Properties
IUPAC Name |
2-(2-hydroxyphenyl)-4,5-dihydro-1,3-thiazole-4-carboxylic acid | |
---|---|---|
Source | PubChem | |
URL | https://pubchem.ncbi.nlm.nih.gov | |
Description | Data deposited in or computed by PubChem | |
InChI |
InChI=1S/C10H9NO3S/c12-8-4-2-1-3-6(8)9-11-7(5-15-9)10(13)14/h1-4,7,12H,5H2,(H,13,14) | |
Source | PubChem | |
URL | https://pubchem.ncbi.nlm.nih.gov | |
Description | Data deposited in or computed by PubChem | |
InChI Key |
CECDPVOEINSAQG-UHFFFAOYSA-N | |
Source | PubChem | |
URL | https://pubchem.ncbi.nlm.nih.gov | |
Description | Data deposited in or computed by PubChem | |
Canonical SMILES |
C1C(N=C(S1)C2=CC=CC=C2O)C(=O)O | |
Source | PubChem | |
URL | https://pubchem.ncbi.nlm.nih.gov | |
Description | Data deposited in or computed by PubChem | |
Molecular Formula |
C10H9NO3S | |
Source | PubChem | |
URL | https://pubchem.ncbi.nlm.nih.gov | |
Description | Data deposited in or computed by PubChem | |
DSSTOX Substance ID |
DTXSID201197921 | |
Record name | 4,5-Dihydro-2-(2-hydroxyphenyl)-4-thiazolecarboxylic acid | |
Source | EPA DSSTox | |
URL | https://comptox.epa.gov/dashboard/DTXSID201197921 | |
Description | DSSTox provides a high quality public chemistry resource for supporting improved predictive toxicology. | |
Molecular Weight |
223.25 g/mol | |
Source | PubChem | |
URL | https://pubchem.ncbi.nlm.nih.gov | |
Description | Data deposited in or computed by PubChem | |
CAS No. |
49608-51-7 | |
Record name | 4,5-Dihydro-2-(2-hydroxyphenyl)-4-thiazolecarboxylic acid | |
Source | CAS Common Chemistry | |
URL | https://commonchemistry.cas.org/detail?cas_rn=49608-51-7 | |
Description | CAS Common Chemistry is an open community resource for accessing chemical information. Nearly 500,000 chemical substances from CAS REGISTRY cover areas of community interest, including common and frequently regulated chemicals, and those relevant to high school and undergraduate chemistry classes. This chemical information, curated by our expert scientists, is provided in alignment with our mission as a division of the American Chemical Society. | |
Explanation | The data from CAS Common Chemistry is provided under a CC-BY-NC 4.0 license, unless otherwise stated. | |
Record name | 2'-(2-Hydroxyphenyl)-2'-thiazoline-4'-carboxylic acid | |
Source | ChemIDplus | |
URL | https://pubchem.ncbi.nlm.nih.gov/substance/?source=chemidplus&sourceid=0049608517 | |
Description | ChemIDplus is a free, web search system that provides access to the structure and nomenclature authority files used for the identification of chemical substances cited in National Library of Medicine (NLM) databases, including the TOXNET system. | |
Record name | 49608-51-7 | |
Source | DTP/NCI | |
URL | https://dtp.cancer.gov/dtpstandard/servlet/dwindex?searchtype=NSC&outputformat=html&searchlist=128295 | |
Description | The NCI Development Therapeutics Program (DTP) provides services and resources to the academic and private-sector research communities worldwide to facilitate the discovery and development of new cancer therapeutic agents. | |
Explanation | Unless otherwise indicated, all text within NCI products is free of copyright and may be reused without our permission. Credit the National Cancer Institute as the source. | |
Record name | 4,5-Dihydro-2-(2-hydroxyphenyl)-4-thiazolecarboxylic acid | |
Source | EPA DSSTox | |
URL | https://comptox.epa.gov/dashboard/DTXSID201197921 | |
Description | DSSTox provides a high quality public chemistry resource for supporting improved predictive toxicology. | |
Retrosynthesis Analysis
AI-Powered Synthesis Planning: Our tool employs the Template_relevance Pistachio, Template_relevance Bkms_metabolic, Template_relevance Pistachio_ringbreaker, Template_relevance Reaxys, Template_relevance Reaxys_biocatalysis model, leveraging a vast database of chemical reactions to predict feasible synthetic routes.
One-Step Synthesis Focus: Specifically designed for one-step synthesis, it provides concise and direct routes for your target compounds, streamlining the synthesis process.
Accurate Predictions: Utilizing the extensive PISTACHIO, BKMS_METABOLIC, PISTACHIO_RINGBREAKER, REAXYS, REAXYS_BIOCATALYSIS database, our tool offers high-accuracy predictions, reflecting the latest in chemical research and data.
Strategy Settings
Precursor scoring | Relevance Heuristic |
---|---|
Min. plausibility | 0.01 |
Model | Template_relevance |
Template Set | Pistachio/Bkms_metabolic/Pistachio_ringbreaker/Reaxys/Reaxys_biocatalysis |
Top-N result to add to graph | 6 |
Feasible Synthetic Routes
Q1: What is Dihydroaeruginoic acid (Dha) and what is its significance in bacterial systems?
A1: this compound (Dha) is a precursor molecule in the biosynthesis of pyochelin, a siderophore produced by Pseudomonas aeruginosa. Siderophores are high-affinity iron-chelating molecules secreted by bacteria to acquire iron from the environment, which is essential for their growth and survival. [, ]. Dha itself has also been shown to bind iron and promote growth in Pseudomonads, suggesting siderophore-like activity [].
Q2: How is Dha biosynthesized in Pseudomonas aeruginosa?
A2: The biosynthesis of Dha in Pseudomonas aeruginosa involves a multi-step enzymatic pathway. The process begins with the activation of salicylic acid by the enzyme PchD, encoded by the pchD gene. PchD, a salicylate-AMP ligase, catalyzes the adenylation of salicylic acid. This activated salicylate molecule then reacts with cysteine, followed by cyclization, to form Dha. This pathway is regulated by iron availability, with iron-depleted conditions inducing the expression of the pchDCBA operon responsible for Dha and pyochelin synthesis [, ].
Q3: Does Dha have any known biological activity apart from its role in pyochelin biosynthesis?
A3: Yes, Dha has been identified as an antifungal antibiotic. Research has shown that it exhibits inhibitory activity against various phytopathogenic fungi and bacteria, including Septoria tritici, a fungal pathogen that causes Septoria leaf blotch in wheat [].
Q4: Are there any studies investigating the structure-activity relationship (SAR) of Dha?
A4: While the provided abstracts don't detail specific SAR studies for Dha, one research group explored high-throughput synthesis approaches to generate Dha analogs [, ]. This suggests an interest in understanding the relationship between Dha's structure and its biological activities, potentially for developing novel antifungal agents or exploring its role in bacterial iron acquisition.
Q5: What is the impact of transporter proteins on Dha production in bacteria?
A5: Studies on Pseudomonas protegens Pf-5, a biocontrol bacterium, reveal that certain transporters encoded within or near the enantio-pyochelin (an enantiomer of pyochelin) biosynthetic gene cluster influence the secretion of Dha and other pyochelin intermediates. For instance, deletion of the pchH and fetF transporter genes resulted in increased secretion of Dha and other intermediates without significantly impacting the transcription of biosynthetic genes. This suggests a role for these transporters in regulating the efflux of these compounds [].
Q6: Are there any known inhibitors of Dha production?
A6: While not explicitly stated in the provided abstracts, the fact that Dha biosynthesis is induced under iron-limiting conditions suggests that iron itself acts as an inhibitor of its production. When iron is abundant, the ferric uptake regulator (Fur) represses the expression of the pchDCBA operon, thereby downregulating Dha synthesis [].
Disclaimer and Information on In-Vitro Research Products
Please be aware that all articles and product information presented on BenchChem are intended solely for informational purposes. The products available for purchase on BenchChem are specifically designed for in-vitro studies, which are conducted outside of living organisms. In-vitro studies, derived from the Latin term "in glass," involve experiments performed in controlled laboratory settings using cells or tissues. It is important to note that these products are not categorized as medicines or drugs, and they have not received approval from the FDA for the prevention, treatment, or cure of any medical condition, ailment, or disease. We must emphasize that any form of bodily introduction of these products into humans or animals is strictly prohibited by law. It is essential to adhere to these guidelines to ensure compliance with legal and ethical standards in research and experimentation.