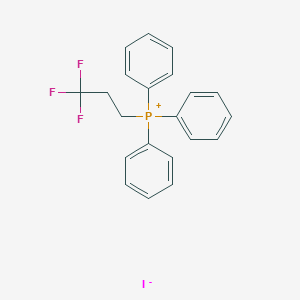
Triphenyl(3,3,3-trifluoropropyl)phosphanium iodide
Overview
Description
Triphenylphosphine is a common organophosphorus compound with the formula P(C6H5)3, often abbreviated to PPh3 or Ph3P . It’s a versatile compound widely used as a reagent in organic synthesis and as a ligand for transition metal complexes .
Synthesis Analysis
Triphenylphosphine can be prepared in the laboratory by treatment of phosphorus trichloride with phenylmagnesium bromide or phenyllithium . The industrial synthesis involves the reaction between phosphorus trichloride, chlorobenzene, and sodium .Molecular Structure Analysis
Triphenylphosphine crystallizes in triclinic and monoclinic modifications . In both cases, the molecule adopts a pyramidal structure with a propeller-like arrangement of the three phenyl groups .Chemical Reactions Analysis
Triphenylphosphine undergoes slow oxidation by air to give triphenylphosphine oxide . Sulfonation of PPh3 gives tris(3-sulfophenyl)phosphine, P(C6H4-3-SO3−)3, usually isolated as the trisodium salt .Physical And Chemical Properties Analysis
Triphenylphosphine is a relatively air-stable, colorless crystal at room temperature . It dissolves in non-polar organic solvents such as benzene and diethyl ether .Scientific Research Applications
Manufacture of Defoaming Agents
The compound (3,3,3-Trifluoropropyl)trimethoxysilane, which has a similar structure to Triphenyl(3,3,3-trifluoropropyl)phosphanium iodide, is used in the manufacture of defoaming agents . It’s plausible that Triphenyl(3,3,3-trifluoropropyl)phosphanium iodide could have similar applications.
Production of Releasing Agents
Similarly, (3,3,3-Trifluoropropyl)trimethoxysilane is used in the production of releasing agents . Given the structural similarities, Triphenyl(3,3,3-trifluoropropyl)phosphanium iodide could potentially be used in a similar manner.
Fluoro Silicone Resin and Coating Production
(3,3,3-Trifluoropropyl)trimethoxysilane is used in the production of fluoro silicone resin and coating . Given the structural similarities, it’s plausible that Triphenyl(3,3,3-trifluoropropyl)phosphanium iodide could be used in similar applications.
Mechanism of Action
Safety and Hazards
Future Directions
properties
IUPAC Name |
triphenyl(3,3,3-trifluoropropyl)phosphanium;iodide | |
---|---|---|
Source | PubChem | |
URL | https://pubchem.ncbi.nlm.nih.gov | |
Description | Data deposited in or computed by PubChem | |
InChI |
InChI=1S/C21H19F3P.HI/c22-21(23,24)16-17-25(18-10-4-1-5-11-18,19-12-6-2-7-13-19)20-14-8-3-9-15-20;/h1-15H,16-17H2;1H/q+1;/p-1 | |
Source | PubChem | |
URL | https://pubchem.ncbi.nlm.nih.gov | |
Description | Data deposited in or computed by PubChem | |
InChI Key |
OILVALOYRFDGPW-UHFFFAOYSA-M | |
Source | PubChem | |
URL | https://pubchem.ncbi.nlm.nih.gov | |
Description | Data deposited in or computed by PubChem | |
Canonical SMILES |
C1=CC=C(C=C1)[P+](CCC(F)(F)F)(C2=CC=CC=C2)C3=CC=CC=C3.[I-] | |
Source | PubChem | |
URL | https://pubchem.ncbi.nlm.nih.gov | |
Description | Data deposited in or computed by PubChem | |
Molecular Formula |
C21H19F3IP | |
Source | PubChem | |
URL | https://pubchem.ncbi.nlm.nih.gov | |
Description | Data deposited in or computed by PubChem | |
DSSTOX Substance ID |
DTXSID00599267 | |
Record name | Triphenyl(3,3,3-trifluoropropyl)phosphanium iodide | |
Source | EPA DSSTox | |
URL | https://comptox.epa.gov/dashboard/DTXSID00599267 | |
Description | DSSTox provides a high quality public chemistry resource for supporting improved predictive toxicology. | |
Molecular Weight |
486.2 g/mol | |
Source | PubChem | |
URL | https://pubchem.ncbi.nlm.nih.gov | |
Description | Data deposited in or computed by PubChem | |
Product Name |
Triphenyl(3,3,3-trifluoropropyl)phosphanium iodide | |
CAS RN |
128622-15-1 | |
Record name | Triphenyl(3,3,3-trifluoropropyl)phosphanium iodide | |
Source | EPA DSSTox | |
URL | https://comptox.epa.gov/dashboard/DTXSID00599267 | |
Description | DSSTox provides a high quality public chemistry resource for supporting improved predictive toxicology. | |
Synthesis routes and methods I
Procedure details
Synthesis routes and methods II
Procedure details
Synthesis routes and methods III
Procedure details
Synthesis routes and methods IV
Procedure details
Disclaimer and Information on In-Vitro Research Products
Please be aware that all articles and product information presented on BenchChem are intended solely for informational purposes. The products available for purchase on BenchChem are specifically designed for in-vitro studies, which are conducted outside of living organisms. In-vitro studies, derived from the Latin term "in glass," involve experiments performed in controlled laboratory settings using cells or tissues. It is important to note that these products are not categorized as medicines or drugs, and they have not received approval from the FDA for the prevention, treatment, or cure of any medical condition, ailment, or disease. We must emphasize that any form of bodily introduction of these products into humans or animals is strictly prohibited by law. It is essential to adhere to these guidelines to ensure compliance with legal and ethical standards in research and experimentation.