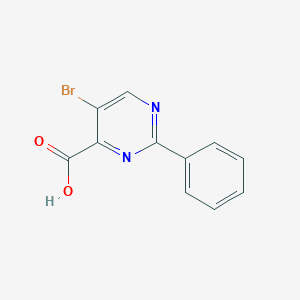
5-Bromo-2-phenylpyrimidine-4-carboxylic acid
Overview
Description
5-Bromo-2-phenylpyrimidine-4-carboxylic acid is a chemical compound with the molecular formula C11H7BrN2O2 and a molecular weight of 279.09 g/mol .
Molecular Structure Analysis
The molecular structure of 5-Bromo-2-phenylpyrimidine-4-carboxylic acid consists of a pyrimidine ring, which is a six-membered ring with two nitrogen atoms, attached to a phenyl group and a carboxylic acid group .Physical And Chemical Properties Analysis
5-Bromo-2-phenylpyrimidine-4-carboxylic acid has a molecular weight of 279.09 g/mol .Scientific Research Applications
1. Chemical Reactions and Mechanisms
5-Bromo-2-phenylpyrimidine-4-carboxylic acid is involved in various chemical reactions and mechanisms. For example, it participates in reactions with potassium amide in liquid ammonia, leading to the formation of 2-amino-4-phenylpyrimidine and related compounds. This process involves a mechanism with an open-chain intermediate (Valk & Plas, 2010). Another study demonstrates the occurrence of the SN(ANRORC)-mechanism in the reaction of 2-bromo-4-phenylpyrimidine with potassium amide (Kroon & Plas, 2010).
2. Liquid-Crystal Characteristics
The compound is also significant in the synthesis of liquid-crystal materials. A study on 5-arylpyrimidine-2-carboxylic acids, synthesized from 5-aryl-2-cyanopyrimidines, revealed their liquid-crystal characteristics, particularly the nematic properties of certain aryl esters (Mikhaleva et al., 1986).
3. Synthesis of Pharmacologically Active Molecules
In pharmacological research, 5-Bromo-2-phenylpyrimidine-4-carboxylic acid is used in the synthesis of active molecules. For instance, its esters have been synthesized via the Minisci homolytic alkoxycarbonylation of 5-halopyrimidines, leading to the creation of CK2 inhibitors (Regan et al., 2012).
4. Luminescent Lanthanide Frameworks
The compound plays a role in developing luminescent materials. Novel luminescent lanthanide-2-phenylpyrimidine-carboxylate frameworks have been synthesized, demonstrating unique photoluminescence behavior (Jia et al., 2014).
5. Antimicrobial and Antifungal Agents
5-Bromo-2-phenylpyrimidine-4-carboxylic acid derivatives exhibit biological activities as antimicrobial and antifungal agents. A study on 5-(2-aminothiazol-4-yl)-3,4-dihydro-4-phenyl pyrimidin-2(1H)-ones showed activity against various microorganisms, indicating its potential in antimicrobial therapy (Lanjewar et al., 2010).
properties
IUPAC Name |
5-bromo-2-phenylpyrimidine-4-carboxylic acid | |
---|---|---|
Source | PubChem | |
URL | https://pubchem.ncbi.nlm.nih.gov | |
Description | Data deposited in or computed by PubChem | |
InChI |
InChI=1S/C11H7BrN2O2/c12-8-6-13-10(14-9(8)11(15)16)7-4-2-1-3-5-7/h1-6H,(H,15,16) | |
Source | PubChem | |
URL | https://pubchem.ncbi.nlm.nih.gov | |
Description | Data deposited in or computed by PubChem | |
InChI Key |
LWLDGZLPOMWNPX-UHFFFAOYSA-N | |
Source | PubChem | |
URL | https://pubchem.ncbi.nlm.nih.gov | |
Description | Data deposited in or computed by PubChem | |
Canonical SMILES |
C1=CC=C(C=C1)C2=NC=C(C(=N2)C(=O)O)Br | |
Source | PubChem | |
URL | https://pubchem.ncbi.nlm.nih.gov | |
Description | Data deposited in or computed by PubChem | |
Molecular Formula |
C11H7BrN2O2 | |
Source | PubChem | |
URL | https://pubchem.ncbi.nlm.nih.gov | |
Description | Data deposited in or computed by PubChem | |
DSSTOX Substance ID |
DTXSID60594399 | |
Record name | 5-Bromo-2-phenylpyrimidine-4-carboxylic acid | |
Source | EPA DSSTox | |
URL | https://comptox.epa.gov/dashboard/DTXSID60594399 | |
Description | DSSTox provides a high quality public chemistry resource for supporting improved predictive toxicology. | |
Molecular Weight |
279.09 g/mol | |
Source | PubChem | |
URL | https://pubchem.ncbi.nlm.nih.gov | |
Description | Data deposited in or computed by PubChem | |
Product Name |
5-Bromo-2-phenylpyrimidine-4-carboxylic acid | |
CAS RN |
179260-95-8 | |
Record name | 5-Bromo-2-phenylpyrimidine-4-carboxylic acid | |
Source | EPA DSSTox | |
URL | https://comptox.epa.gov/dashboard/DTXSID60594399 | |
Description | DSSTox provides a high quality public chemistry resource for supporting improved predictive toxicology. | |
Retrosynthesis Analysis
AI-Powered Synthesis Planning: Our tool employs the Template_relevance Pistachio, Template_relevance Bkms_metabolic, Template_relevance Pistachio_ringbreaker, Template_relevance Reaxys, Template_relevance Reaxys_biocatalysis model, leveraging a vast database of chemical reactions to predict feasible synthetic routes.
One-Step Synthesis Focus: Specifically designed for one-step synthesis, it provides concise and direct routes for your target compounds, streamlining the synthesis process.
Accurate Predictions: Utilizing the extensive PISTACHIO, BKMS_METABOLIC, PISTACHIO_RINGBREAKER, REAXYS, REAXYS_BIOCATALYSIS database, our tool offers high-accuracy predictions, reflecting the latest in chemical research and data.
Strategy Settings
Precursor scoring | Relevance Heuristic |
---|---|
Min. plausibility | 0.01 |
Model | Template_relevance |
Template Set | Pistachio/Bkms_metabolic/Pistachio_ringbreaker/Reaxys/Reaxys_biocatalysis |
Top-N result to add to graph | 6 |
Feasible Synthetic Routes
Q & A
Q1: What happens when 5-Bromo-2-phenylpyrimidine-4-carboxylic acid is subjected to the Busch reaction?
A1: Interestingly, the Busch reaction, typically used to synthesize biaryl compounds, doesn't yield the expected product when applied to 5-Bromo-2-phenylpyrimidine-4-carboxylic acid. Instead of forming a biaryl, the reaction leads to the formation of 2-phenylpyrimidine-4-carboxylic acid. This suggests that the reaction conditions promote debromination rather than the desired coupling reaction. []
Q2: Besides the Busch reaction, is there another way to synthesize 2-phenylpyrimidine-4-carboxylic acid?
A2: Yes, the paper describes an alternative synthesis route for 2-phenylpyrimidine-4-carboxylic acid. This method involves the oxidation of 4-methyl-2-phenylpyrimidine. This finding highlights that different synthetic strategies can be employed to obtain the same target compound. []
Disclaimer and Information on In-Vitro Research Products
Please be aware that all articles and product information presented on BenchChem are intended solely for informational purposes. The products available for purchase on BenchChem are specifically designed for in-vitro studies, which are conducted outside of living organisms. In-vitro studies, derived from the Latin term "in glass," involve experiments performed in controlled laboratory settings using cells or tissues. It is important to note that these products are not categorized as medicines or drugs, and they have not received approval from the FDA for the prevention, treatment, or cure of any medical condition, ailment, or disease. We must emphasize that any form of bodily introduction of these products into humans or animals is strictly prohibited by law. It is essential to adhere to these guidelines to ensure compliance with legal and ethical standards in research and experimentation.