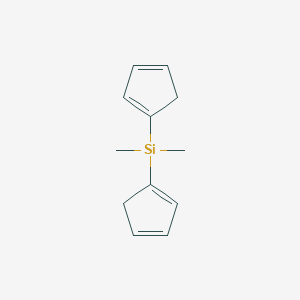
DI(Cyclopentadienyl)dimethylsilane
Overview
Description
DI(Cyclopentadienyl)dimethylsilane is an organosilicon compound with the molecular formula C12H16Si. It is characterized by the presence of two cyclopentadienyl rings attached to a silicon atom, which is also bonded to two methyl groups. This compound is of significant interest in organometallic chemistry due to its unique structure and reactivity.
Preparation Methods
Synthetic Routes and Reaction Conditions
The synthesis of DI(Cyclopentadienyl)dimethylsilane typically involves the reaction of cyclopentadienyl sodium with dimethyldichlorosilane. The reaction is carried out in an inert atmosphere, often using solvents like tetrahydrofuran (THF) to facilitate the reaction. The general reaction scheme is as follows:
2C5H5Na+SiCl2(CH3)2→(C5H5)2Si(CH3)2+2NaCl
Industrial Production Methods
Industrial production methods for this compound are similar to laboratory synthesis but are scaled up to accommodate larger quantities. The reaction conditions are optimized for higher yields and purity, often involving continuous flow reactors and advanced purification techniques.
Chemical Reactions Analysis
Types of Reactions
DI(Cyclopentadienyl)dimethylsilane undergoes various types of chemical reactions, including:
Oxidation: The compound can be oxidized to form silanols or siloxanes.
Reduction: Reduction reactions can lead to the formation of silyl anions.
Substitution: The methyl groups or cyclopentadienyl rings can be substituted with other functional groups.
Common Reagents and Conditions
Oxidation: Common oxidizing agents include hydrogen peroxide (H2O2) and potassium permanganate (KMnO4).
Reduction: Reducing agents such as lithium aluminum hydride (LiAlH4) are often used.
Substitution: Substitution reactions may involve reagents like Grignard reagents or organolithium compounds.
Major Products
The major products formed from these reactions depend on the specific conditions and reagents used. For example, oxidation can yield silanols, while substitution reactions can produce a variety of organosilicon compounds.
Scientific Research Applications
DI(Cyclopentadienyl)dimethylsilane has a wide range of applications in scientific research:
Chemistry: It is used as a precursor for the synthesis of various organosilicon compounds and as a ligand in transition metal complexes.
Biology: Research is ongoing into its potential use in drug delivery systems due to its ability to form stable complexes with metals.
Medicine: Its derivatives are being explored for their potential use in medical imaging and as therapeutic agents.
Industry: It is used in the production of high-performance materials, including polymers and coatings.
Mechanism of Action
The mechanism by which DI(Cyclopentadienyl)dimethylsilane exerts its effects is primarily through its ability to form stable complexes with transition metals. The cyclopentadienyl rings act as ligands, coordinating with metal centers to form metallocenes. These complexes are highly stable and exhibit unique reactivity, making them valuable in catalysis and other applications.
Comparison with Similar Compounds
Similar Compounds
Ferrocene: (C5H5)2Fe
Ruthenocene: (C5H5)2Ru
Zirconocene: (C5H5)2ZrCl2
Uniqueness
DI(Cyclopentadienyl)dimethylsilane is unique due to the presence of silicon, which imparts different chemical properties compared to its carbon-based analogs. The silicon atom allows for greater flexibility in the types of reactions the compound can undergo, making it a versatile building block in organometallic chemistry.
Properties
IUPAC Name |
di(cyclopenta-1,3-dien-1-yl)-dimethylsilane | |
---|---|---|
Source | PubChem | |
URL | https://pubchem.ncbi.nlm.nih.gov | |
Description | Data deposited in or computed by PubChem | |
InChI |
InChI=1S/C12H16Si/c1-13(2,11-7-3-4-8-11)12-9-5-6-10-12/h3-7,9H,8,10H2,1-2H3 | |
Source | PubChem | |
URL | https://pubchem.ncbi.nlm.nih.gov | |
Description | Data deposited in or computed by PubChem | |
InChI Key |
FPWYHHNBOYUKSS-UHFFFAOYSA-N | |
Source | PubChem | |
URL | https://pubchem.ncbi.nlm.nih.gov | |
Description | Data deposited in or computed by PubChem | |
Canonical SMILES |
C[Si](C)(C1=CC=CC1)C2=CC=CC2 | |
Source | PubChem | |
URL | https://pubchem.ncbi.nlm.nih.gov | |
Description | Data deposited in or computed by PubChem | |
Molecular Formula |
C12H16Si | |
Source | PubChem | |
URL | https://pubchem.ncbi.nlm.nih.gov | |
Description | Data deposited in or computed by PubChem | |
DSSTOX Substance ID |
DTXSID30478233 | |
Record name | Silane, dicyclopentadienyldimethyl- | |
Source | EPA DSSTox | |
URL | https://comptox.epa.gov/dashboard/DTXSID30478233 | |
Description | DSSTox provides a high quality public chemistry resource for supporting improved predictive toxicology. | |
Molecular Weight |
188.34 g/mol | |
Source | PubChem | |
URL | https://pubchem.ncbi.nlm.nih.gov | |
Description | Data deposited in or computed by PubChem | |
CAS No. |
107241-50-9 | |
Record name | Silane, dicyclopentadienyldimethyl- | |
Source | EPA DSSTox | |
URL | https://comptox.epa.gov/dashboard/DTXSID30478233 | |
Description | DSSTox provides a high quality public chemistry resource for supporting improved predictive toxicology. | |
Retrosynthesis Analysis
AI-Powered Synthesis Planning: Our tool employs the Template_relevance Pistachio, Template_relevance Bkms_metabolic, Template_relevance Pistachio_ringbreaker, Template_relevance Reaxys, Template_relevance Reaxys_biocatalysis model, leveraging a vast database of chemical reactions to predict feasible synthetic routes.
One-Step Synthesis Focus: Specifically designed for one-step synthesis, it provides concise and direct routes for your target compounds, streamlining the synthesis process.
Accurate Predictions: Utilizing the extensive PISTACHIO, BKMS_METABOLIC, PISTACHIO_RINGBREAKER, REAXYS, REAXYS_BIOCATALYSIS database, our tool offers high-accuracy predictions, reflecting the latest in chemical research and data.
Strategy Settings
Precursor scoring | Relevance Heuristic |
---|---|
Min. plausibility | 0.01 |
Model | Template_relevance |
Template Set | Pistachio/Bkms_metabolic/Pistachio_ringbreaker/Reaxys/Reaxys_biocatalysis |
Top-N result to add to graph | 6 |
Feasible Synthetic Routes
Q1: What is the role of di(cyclopentadienyl)dimethylsilane in synthesizing ruthenocenophanes?
A1: this compound serves as a crucial precursor in the synthesis of [1.1]ruthenocenophanes, specifically bis(dimethylsila)-[1.1]ruthenocenophane []. The synthesis involves reacting dilithium this compound with RuCl2·4 dmso in a tetrahydrofuran solution []. This reaction highlights the compound's ability to act as a ligand source, contributing both cyclopentadienyl rings and the bridging dimethylsilyl group to the final ruthenocenophane structure.
Disclaimer and Information on In-Vitro Research Products
Please be aware that all articles and product information presented on BenchChem are intended solely for informational purposes. The products available for purchase on BenchChem are specifically designed for in-vitro studies, which are conducted outside of living organisms. In-vitro studies, derived from the Latin term "in glass," involve experiments performed in controlled laboratory settings using cells or tissues. It is important to note that these products are not categorized as medicines or drugs, and they have not received approval from the FDA for the prevention, treatment, or cure of any medical condition, ailment, or disease. We must emphasize that any form of bodily introduction of these products into humans or animals is strictly prohibited by law. It is essential to adhere to these guidelines to ensure compliance with legal and ethical standards in research and experimentation.