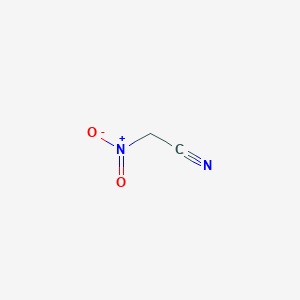
Nitroacetonitrile
Overview
Description
Nitroacetonitrile is the simplest α-nitronitrile, characterized by a single central carbon atom attached to two strongly electronegative, electron-withdrawing groups: a nitro group and a nitrile group . This unique structure allows for extensive chemistry through the active methylene center, making it a versatile precursor in various synthetic applications .
Preparation Methods
Synthetic Routes and Reaction Conditions: Nitroacetonitrile can be synthesized through several methods. One common approach involves the reaction of sodium nitrite with acetonitrile in the presence of an acid, forming this compound . Another method includes the use of dipyrrolidinium cyano-aci-nitroacetate, which is soluble in common organic solvents and thermally stable, allowing for its use as an alternative synthetic equivalent of this compound .
Industrial Production Methods: In industrial settings, this compound is often produced by the nitration of acetonitrile using nitric acid and sulfuric acid under controlled conditions . The resulting product is then purified to obtain this compound in its pure form.
Chemical Reactions Analysis
Types of Reactions: Nitroacetonitrile undergoes various chemical reactions, including:
Oxidation: this compound can be oxidized to form different products depending on the oxidizing agent used.
Reduction: Reduction of this compound typically leads to the formation of aminoacetonitrile.
Substitution: this compound can participate in substitution reactions, where the nitro or nitrile group is replaced by other functional groups.
Common Reagents and Conditions:
Oxidation: Hypofluorous acid in acetonitrile.
Reduction: Hydrogenation using palladium on carbon as a catalyst.
Substitution: Reactions with aromatic and heterocyclic aldehydes to form 3-(het)aryl-2-nitroacrylonitriles.
Major Products:
Oxidation: 4-amino-3,7-dinitrotriazolo-[5,1-c][1,2,4]triazine 4-oxide.
Reduction: Aminoacetonitrile.
Substitution: 3-(het)aryl-2-nitroacrylonitriles.
Scientific Research Applications
Nitroacetonitrile serves as a versatile synthetic precursor in the formation of heterocyclic and polyfunctional aliphatic products . It allows for straightforward conversion to amino, acyl, and other functional groups, making it valuable in the synthesis of heterocyclic-based energetics . Additionally, this compound is used in the development of new energetic materials due to its ability to add vicinal amino and nitro moieties onto fused ring structures .
Mechanism of Action
The mechanism of action of nitroacetonitrile involves its role as a cyano(nitro)methylation agent . The compound’s central carbon, flanked by the nitro and nitrile groups, exhibits increased acidity, allowing for the generation of resonance-stabilized nucleophiles . These nucleophiles can participate in various chemical transformations, leading to the formation of polyfunctionalized compounds .
Comparison with Similar Compounds
Malononitrile: Similar in that it contains a nitrile group, but lacks the nitro group.
Cyanoacetate: Contains a nitrile group and an ester group, offering different reactivity compared to nitroacetonitrile.
Nitroacetate: Contains a nitro group and an ester group, providing different chemical properties.
Uniqueness: this compound’s uniqueness lies in its combination of a nitro group and a nitrile group attached to the same carbon atom, allowing for extensive chemistry through the active methylene center . This dual functionality makes it a valuable precursor in the synthesis of complex heterocyclic compounds and energetic materials .
Properties
IUPAC Name |
2-nitroacetonitrile | |
---|---|---|
Source | PubChem | |
URL | https://pubchem.ncbi.nlm.nih.gov | |
Description | Data deposited in or computed by PubChem | |
InChI |
InChI=1S/C2H2N2O2/c3-1-2-4(5)6/h2H2 | |
Source | PubChem | |
URL | https://pubchem.ncbi.nlm.nih.gov | |
Description | Data deposited in or computed by PubChem | |
InChI Key |
DWBOSISZPCOPFS-UHFFFAOYSA-N | |
Source | PubChem | |
URL | https://pubchem.ncbi.nlm.nih.gov | |
Description | Data deposited in or computed by PubChem | |
Canonical SMILES |
C(C#N)[N+](=O)[O-] | |
Source | PubChem | |
URL | https://pubchem.ncbi.nlm.nih.gov | |
Description | Data deposited in or computed by PubChem | |
Molecular Formula |
C2H2N2O2 | |
Source | PubChem | |
URL | https://pubchem.ncbi.nlm.nih.gov | |
Description | Data deposited in or computed by PubChem | |
DSSTOX Substance ID |
DTXSID40902418 | |
Record name | NoName_1661 | |
Source | EPA DSSTox | |
URL | https://comptox.epa.gov/dashboard/DTXSID40902418 | |
Description | DSSTox provides a high quality public chemistry resource for supporting improved predictive toxicology. | |
Molecular Weight |
86.05 g/mol | |
Source | PubChem | |
URL | https://pubchem.ncbi.nlm.nih.gov | |
Description | Data deposited in or computed by PubChem | |
Retrosynthesis Analysis
AI-Powered Synthesis Planning: Our tool employs the Template_relevance Pistachio, Template_relevance Bkms_metabolic, Template_relevance Pistachio_ringbreaker, Template_relevance Reaxys, Template_relevance Reaxys_biocatalysis model, leveraging a vast database of chemical reactions to predict feasible synthetic routes.
One-Step Synthesis Focus: Specifically designed for one-step synthesis, it provides concise and direct routes for your target compounds, streamlining the synthesis process.
Accurate Predictions: Utilizing the extensive PISTACHIO, BKMS_METABOLIC, PISTACHIO_RINGBREAKER, REAXYS, REAXYS_BIOCATALYSIS database, our tool offers high-accuracy predictions, reflecting the latest in chemical research and data.
Strategy Settings
Precursor scoring | Relevance Heuristic |
---|---|
Min. plausibility | 0.01 |
Model | Template_relevance |
Template Set | Pistachio/Bkms_metabolic/Pistachio_ringbreaker/Reaxys/Reaxys_biocatalysis |
Top-N result to add to graph | 6 |
Feasible Synthetic Routes
Disclaimer and Information on In-Vitro Research Products
Please be aware that all articles and product information presented on BenchChem are intended solely for informational purposes. The products available for purchase on BenchChem are specifically designed for in-vitro studies, which are conducted outside of living organisms. In-vitro studies, derived from the Latin term "in glass," involve experiments performed in controlled laboratory settings using cells or tissues. It is important to note that these products are not categorized as medicines or drugs, and they have not received approval from the FDA for the prevention, treatment, or cure of any medical condition, ailment, or disease. We must emphasize that any form of bodily introduction of these products into humans or animals is strictly prohibited by law. It is essential to adhere to these guidelines to ensure compliance with legal and ethical standards in research and experimentation.