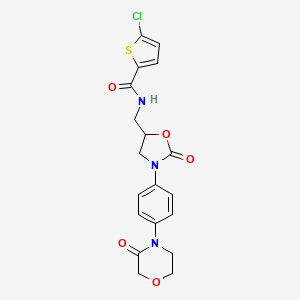
Rivaroxaban
Overview
Description
Rivaroxaban is a direct oral Factor Xa (FXa) inhibitor approved for the prevention and treatment of thromboembolic disorders, including venous thromboembolism (VTE), stroke in nonvalvular atrial fibrillation (NVAF), and acute coronary syndromes (ACS). It binds selectively and reversibly to FXa, interrupting the coagulation cascade and reducing thrombin generation. This compound has a high bioavailability (>80% for doses ≤10 mg) and rapid absorption (peak plasma concentration within 1.25–4.0 hours post-dose), with dose-dependent pharmacokinetics influenced by food intake for higher doses (15–20 mg) . Approximately two-thirds of the drug undergoes hepatic metabolism via CYP3A4/2J2 and CYP-independent pathways, with renal and fecal excretion .
Preparation Methods
Chemical Structure and Properties
Rivaroxaban (C₁₉H₁₈ClN₃O₅S) has a molecular weight of 435.88 and exists as an odorless, non-hygroscopic, white to yellowish powder. It is only slightly soluble in organic solvents such as acetone and polyethylene glycol 400, and is practically insoluble in water and aqueous media. The compound contains a critical chiral center, requiring stereoselective synthetic approaches to ensure the correct configuration is maintained throughout the preparation process.
Classical Synthetic Routes
Original Patented Process
The seminal preparation method for this compound was first disclosed in patent EP 1261606, proposing a synthesis through the reaction between 4-(4-aminophenyl)morpholin-3-one and terminal epoxides such as 2-((oxiran-2-yl)methyl)isoindoline-1,3-dione or 5-chloro-N-((oxiran-2-yl)methyl)thiophene-2-carboxamide, followed by oxazolidinone formation.
A more refined process was later detailed in patent WO2015198259A1, outlining a four-step synthesis as illustrated below:
Step a: Reaction of 4-(4-aminophenyl)morpholine-3-one (compound I) with (S)-(+)-N(2,3-epoxypropyl)phthalimide (compound II) to yield 2-{(R)-2-hydroxy-3-[4-(3-oxo-morpholin-4-yl)phenylamino]propyl}isoindol-1,3-dione (compound III).
Step b: Reaction of compound III with N,N-carbonyldiimidazole (CDI) to form the cyclic carbamate, producing 2-{(S)-2-oxo-3-[4-(3-oxo-morpholin-4-yl)phenyl]-oxazolidin-5-yl-methyl}-isoindol-1,3-dione (compound IV).
Step c: Reaction of compound IV with methylamine followed by nitric acid addition to generate (5S)-4-{4-[5-(aminomethyl)-2-oxo-1,3-oxazolidin-3-yl]phenyl}morpholin-3-one nitrate (compound V).
Step d: Final reaction of compound V with 5-chlorothiophene-2-carbonyl chloride to yield this compound.
The reaction conditions for these steps are meticulously controlled:
In step a, methanol serves as the solvent, with compound II used in slight excess (molar ratio 1.2-1.3). The reaction proceeds at 50-65°C for 15-25 hours, after which the precipitated product is filtered and dried.
For step b, CDI is employed in slight excess (molar ratio 1.2-1.3), with chlorobenzene as the preferred solvent. The reaction runs at approximately 80°C for several hours, followed by cooling, filtration, and drying.
In step c, the isolation of compound V as its nitrate salt represents a key innovation, providing a stable intermediate that enhances the overall process efficiency.
The final step d involves reacting compound V with 5-chlorothiophene-2-carbonyl chloride (prepared in situ from 5-chloro-2-thiophenecarboxylic acid and thionyl chloride) at temperatures between -5°C and 35°C in the presence of triethylamine. The reaction completes in approximately one hour, and water addition creates a biphasic system from which the product is isolated.
Key Intermediate Approaches
Alternative approaches to key intermediates have been developed to optimize the synthesis process. A critical intermediate is 4-(4-((S)-5-(aminomethyl)-2-oxooxazolidin-3-yl)phenyl)morpholin-3-one, which can be prepared through multiple routes:
Transformation of an alcohol precursor to a mesylate, followed by conversion to an amine through:
- Formation and reduction of an azide
- Displacement with potassium phthalimide followed by deprotection
- Direct reaction with ammonium hydroxide in a sealed system at 270 kPa pressure
Novel key intermediates like 4-{4-[(SS)-(Aminomethyl)-2-oxo-1,3-oxazolidin-3-yl]-phenyl}-morpholin-3-one perchlorate have been developed, offering improved efficiency in this compound preparation.
Alternative Synthetic Methods
Novel Starting Materials
Patent CN-105801572-A details an innovative preparation method using (S)-4-chloro-3-hydroxybutyronitrile as the starting material instead of the more hazardous (S)-epichlorohydrin traditionally used to introduce the chiral center. This pathway involves:
- Substitution with phthalimide potassium salt
- Hydrolysis of the nitrile group
- Hofmann rearrangement ring closure
- Ullmann coupling
- Hydrazinolysis
- Amidation to yield this compound
This alternative method eliminates the need for expensive catalysts and environmentally problematic reagents, resulting in a process with reduced pollution, easier handling, high yields, and lower production costs, making it particularly suitable for industrial scale manufacturing.
Improved Salt Strategies
The identification of stable salt forms of key intermediates has significantly advanced this compound synthesis efficiency. The use of the nitrate salt of (5S)-4-{4-[5-(aminomethyl)-2-oxo-1,3-oxazolidin-3-yl]phenyl}morpholin-3-one (Compound V) has been particularly impactful. This approach offers several advantages:
- Enhanced stability of the intermediate
- Improved isolation efficiency
- Higher purity of the final this compound product
Similarly, the perchlorate salt approach described in patent literature provides an alternative pathway with its own efficiency benefits.
Formulation Techniques
Solid Solution Formulations
Two primary approaches for developing immediate-release formulations with reliable dissolution properties have been documented:
Table 1: Solid Solution Formulation Approaches for this compound
Parameter | HPMCP Prototype | Kollidon Prototype |
---|---|---|
Methodology | Spray-drying process | Hot melt extrusion |
Composition ratio | 1:3 (this compound:HPMCP) | 1:4.5:0.055 (this compound:Kollidon VA64:Polysorbate 80) |
Primary solvent | 2,2,2-trifluoroethanol | N/A (solvent-free process) |
Process temperature | Inlet: 90-100°C | 140-200°C (heating segments) |
Processing rate | Feed rate: 1 kg/h | Feed rate: 200 g/h |
Other parameters | Air atomization: 40 m³/h, Aspiration: 85% | Screws speed: 50 rpm |
Post-processing | Vacuum drying at 45°C | Milling and homogenization |
Both methods culminate in homogenization with excipients including lactose monohydrate, sodium cross carmellose, sodium lauryl sulfate, silicified cellulose, and sodium stearyl fumarate, followed by compaction to obtain a granulate suitable for tablet formation.
Crystallization Processes
A specialized crystallization process has been developed for producing the this compound-oxalic acid cocrystal, which enhances solubility and dissolution characteristics. This process involves:
- Solvent screening with acetone, ethanol, isopropanol, acetonitrile, ethyl acetate, and ethyl formate
- Construction of ternary phase diagrams for ethyl formate and acetone to determine system equilibrium conditions
- Process monitoring using in situ Raman spectroscopy
- Crystallization mechanism analysis via imaging probe technology
The research determined that ethyl formate is the optimal solvent for scale-up, delivering more favorable dissolution enhancement despite acetone producing larger crystals.
Dosage Forms and Clinical Applications
This compound is primarily administered as film-coated tablets in various strengths according to the clinical indication. For coronary artery disease (CAD) or peripheral artery disease (PAD), a 2.5 mg oral dose twice daily is recommended, in combination with aspirin (75 to 100 mg) once daily.
Table 2: this compound Treatment Dosing by Weight for Pediatric Patients
Weight | Dose | Dosing Interval |
---|---|---|
2.6 to 2.9 kg | 0.8 mg/dose | Q8 hours |
3 to 3.9 kg | 0.9 mg/dose | Q8 hours |
4 to 4.9 kg | 1.4 mg/dose | Q8 hours |
5 to 6.9 kg | 1.6 mg/dose | Q8 hours |
7 to 7.9 kg | 1.8 mg/dose | Q8 hours |
8 to 8.9 kg | 2.4 mg/dose | Q8 hours |
9 to 9.9 kg | 2.8 mg/dose | Q8 hours |
10 to 11.9 kg | 3 mg/dose | Q8 hours |
12 to 29.9 kg | 5 mg/dose | Q12 hours |
30 to 49.9 kg | 15 mg/dose | Q24 hours |
≥50 kg | 20 mg/dose | Q24 hours |
For prophylaxis, modified dosing schedules are employed based on patient weight and age.
Industrial Production Considerations
Scale-up Challenges
Several challenges arise when scaling this compound synthesis to industrial levels:
Preparation of unstable intermediates, particularly 5-chlorothiophene-2-carbonyl chloride, which must be generated in situ shortly before use
Maintaining stereochemical purity throughout the multi-step process, which is critical for pharmacological activity
Implementing effective purification strategies for pharmaceutical-grade output while maintaining economic viability
Comparative Analysis of Preparation Methods
Efficiency and Yield Comparison
The various preparation methods offer different advantages in terms of efficiency and overall yield:
The classical nitrate salt intermediate route described in WO2015198259A1 provides good yields with well-established protocols suitable for industrial production
The alternative approach utilizing (S)-4-chloro-3-hydroxybutyronitrile claims high yields at each synthetic step with simplified processing requirements
Formulation approaches like HPMCP spray-drying and Kollidon hot melt extrusion represent different efficiency trade-offs depending on available equipment and specific product requirements
Purity and Polymorphism Considerations
The purity of this compound is paramount for pharmaceutical applications:
The nitrate salt intermediate route produces high-purity this compound as the salt formation helps eliminate impurities during isolation
The this compound-oxalic acid cocrystal approach enhances both solubility and purity through controlled crystallization processes
When produced by these optimized processes, this compound typically maintains the same polymorphic form (Form I) as originally described, confirmed by X-ray powder diffraction analysis
Pharmacokinetic Implications
The preparation method can significantly impact the pharmacokinetic profile of the final drug product:
Solid solution formulations enhance bioavailability by improving dissolution characteristics, particularly important given this compound's low aqueous solubility
The cocrystal approach with oxalic acid provides notable enhancement in solubility and dissolution parameters, potentially improving therapeutic efficacy
These formulation strategies support this compound's predictable pharmacodynamics with both once- and twice-daily dosing regimens, as demonstrated in clinical studies
Chemical Reactions Analysis
Types of Reactions: Rivaroxaban undergoes various chemical reactions, including oxidation, reduction, and substitution.
Common Reagents and Conditions:
Oxidation: Involves the use of oxidizing agents such as hydrogen peroxide or potassium permanganate.
Reduction: Typically employs reducing agents like lithium aluminum hydride or sodium borohydride.
Substitution: Often uses reagents like thionyl chloride for the formation of acid chlorides.
Major Products Formed: The major products formed from these reactions include intermediates such as acid chlorides, amides, and oxazolidinones, which are crucial for the synthesis of this compound .
Scientific Research Applications
Indications for Use
Rivaroxaban is indicated for several clinical conditions, including:
- Treatment of Venous Thromboembolism (VTE) : this compound is used to treat deep vein thrombosis and pulmonary embolism.
- Prevention of VTE : It is prescribed to prevent VTE recurrence in patients who have undergone orthopedic surgeries such as knee or hip replacements.
- Atrial Fibrillation : this compound is indicated for the prevention of stroke and systemic embolism in patients with nonvalvular atrial fibrillation.
- Coronary Artery Disease : Recent studies suggest its use in patients with coronary artery disease to reduce cardiovascular events when combined with antiplatelet therapy.
Treatment of VTE
The EINSTEIN trials demonstrated the efficacy of this compound in treating VTE. In these studies, this compound was found to be as effective as conventional therapies (such as low molecular weight heparin followed by vitamin K antagonists) with a comparable safety profile.
Study | Population | Treatment | Outcome |
---|---|---|---|
EINSTEIN DVT | Patients with DVT | This compound vs. Enoxaparin/VKA | Non-inferiority demonstrated |
EINSTEIN PE | Patients with PE | This compound vs. Enoxaparin/VKA | Non-inferiority demonstrated |
Atrial Fibrillation
This compound is extensively used in patients with nonvalvular atrial fibrillation to prevent stroke. A meta-analysis indicated that this compound significantly reduces the risk of stroke compared to placebo and has a favorable bleeding profile.
Study | Population | Treatment | Efficacy Endpoint |
---|---|---|---|
ROCKET AF | Patients with AF | This compound vs. Warfarin | Reduced stroke rate (1.7% vs 2.2%) |
Cardiovascular Disease
Recent studies have explored low-dose this compound (2.5 mg twice daily) in combination with antiplatelet therapy for secondary prevention in patients with coronary artery disease and peripheral artery disease.
Study | Population | Treatment | Outcome |
---|---|---|---|
COMPASS Trial | Patients with CAD/PAD | Low-dose this compound + Aspirin vs. Aspirin alone | Significant reduction in cardiovascular events |
Case Studies
Several case studies have highlighted both the efficacy and potential adverse effects associated with this compound:
- A case study reported successful treatment of a patient with pulmonary embolism using this compound, showcasing its effectiveness in acute settings.
- Conversely, another case questioned its efficacy in treating left ventricular thrombus, suggesting that more research is needed to establish its role in such conditions.
Adverse Effects and Considerations
While this compound is generally well-tolerated, it is not without risks. Common adverse effects include bleeding complications, which are critical to monitor, especially in elderly patients or those with renal impairment. A review of adverse drug reactions reported significant instances of serious bleeding events associated with this compound usage.
Mechanism of Action
Rivaroxaban exerts its effects by competitively inhibiting both free and clot-bound Factor Xa. Factor Xa is essential for the activation of prothrombin to thrombin, a key step in the blood clotting cascade . By inhibiting Factor Xa, this compound effectively reduces the formation of blood clots .
Comparison with Similar Compounds
Rivaroxaban is part of the direct oral anticoagulant (DOAC) class, which includes dabigatran (a thrombin inhibitor) and other FXa inhibitors (apixaban, edoxaban, betrixaban ). Key comparisons are outlined below:
This compound vs. Warfarin
- Efficacy: this compound demonstrated non-inferiority to warfarin in stroke prevention (ROCKET-AF trial) and VTE treatment (EINSTEIN trials), with comparable rates of recurrent VTE (HR 0.89, 95% CI 0.66–1.19) . In cerebral venous sinus thrombosis (CVST), this compound showed similar clinical benefit (100% recanalization rate in small studies) but faster recovery times compared to warfarin .
- Safety : Major bleeding rates were similar (3.6% vs. 3.4% annually), but this compound reduced intracranial hemorrhage risk (0.5% vs. 0.7%) .
This compound vs. Dabigatran
- Efficacy : In NVAF, this compound (20 mg) and dabigatran (150 mg) showed comparable stroke prevention (HR 1.03, 95% CI 0.84–1.27), but this compound had higher gastrointestinal bleeding risk (3.2% vs. 2.2%) .
- Mortality : A Danish registry study found this compound associated with higher all-cause mortality vs. dabigatran (HR 1.43, 95% CI 1.13–1.81) .
- Pharmacokinetics : this compound’s renal clearance (35%) is lower than dabigatran’s (80%), making it preferable in mild renal impairment .
This compound vs. Apixaban
- Efficacy : In the GLORIA-AF registry, this compound and apixaban had similar stroke risks (HR 0.78, 95% CI 0.52–1.19) .
- Safety : this compound had a 54% higher major bleeding risk vs. apixaban (HR 1.54, 95% CI 1.14–2.08), attributed to apixaban’s twice-daily dosing and lower peak concentrations .
- Thrombin Inhibition : this compound caused stronger suppression of peak thrombin (8.1 nM vs. 13.6 nM for apixaban) in plasma-based assays .
This compound vs. Edoxaban and Betrixaban
- FXa Inhibition: this compound showed the most pronounced effects on thrombin generation lag time (delayed clotting initiation) compared to edoxaban and betrixaban .
- Anticoagulant Potency : In whole-blood assays, this compound’s effects were milder than edoxaban but stronger than betrixaban in plasma .
This compound vs. Aspirin
- Thromboprophylaxis : Post-lower extremity revascularization, this compound + aspirin reduced ischemic events (HR 0.76, 95% CI 0.62–0.95) vs. aspirin alone, with similar bleeding rates .
- Mechanism : Aspirin’s antiplatelet action complements this compound’s anticoagulant effects, though dual therapy increases bleeding risk in ACS .
Data Tables
Table 2. Pharmacokinetic and Pharmacodynamic Profiles
Parameter | This compound | Apixaban | Edoxaban | Betrixaban |
---|---|---|---|---|
Bioavailability (%) | >80 | 50 | 62 | 34 |
Half-life (hours) | 5–13 | 8–15 | 9–11 | 19–27 |
Renal Clearance (%) | 35 | 27 | 50 | 5–7 |
Peak Thrombin (nM) | 8.1 | 13.6 | 9.6 | 12.5 |
Key Clinical Findings
Renal Impairment : this compound’s efficacy and safety are comparable to warfarin in mild-to-moderate renal impairment but require dose adjustment (15 mg daily) in severe cases .
Drug Interactions : Strong CYP3A4/P-gp inhibitors (e.g., clarithromycin) increase this compound exposure by 1.5–2.0-fold, necessitating dose reduction .
Reversal Agents : Andexanet alfa is approved for this compound reversal, with prothrombin complex concentrates used off-label .
Biological Activity
Rivaroxaban is a direct oral anticoagulant (DOAC) that selectively inhibits factor Xa, playing a crucial role in the management of thromboembolic disorders. Its biological activity extends beyond simple anticoagulation, influencing various biological pathways, including inflammation and endothelial function. This article provides a detailed overview of this compound's biological activity, supported by data tables, case studies, and significant research findings.
This compound exerts its anticoagulant effect through the direct and specific inhibition of factor Xa. This inhibition disrupts the coagulation cascade, leading to reduced thrombin generation and subsequent clot formation. Key findings from preclinical studies indicate that:
- Inhibition Constant : this compound has a low inhibition constant (K_i), demonstrating high binding affinity to factor Xa.
- Thrombin Generation : In whole blood and platelet-rich plasma, this compound significantly prolongs the initiation phase of thrombin generation and reduces endogenous thrombin potential (ETP) at nanomolar concentrations .
Pharmacodynamic Profile
The pharmacodynamic profile of this compound has been extensively characterized through various studies:
Study | Concentration | Effect on ETP | Thrombin Generation |
---|---|---|---|
Gerotziafas et al. (2007) | 35 nM | 50% reduction | Almost complete inhibition at 80-100 nM |
These results indicate that this compound effectively modulates coagulation parameters even at low concentrations, making it a potent anticoagulant agent.
Clinical Trials and Efficacy
The EINSTEIN clinical trial program provided robust evidence for the efficacy and safety of this compound in treating venous thromboembolism (VTE). Key outcomes from these trials include:
- EINSTEIN DVT and PE Trials : this compound was shown to be an effective alternative to standard anticoagulation treatments for deep vein thrombosis (DVT) and pulmonary embolism (PE), with a dosing regimen of 15 mg twice daily followed by 20 mg once daily .
- EINSTEIN EXT Trial : Demonstrated that this compound is superior to placebo for extended treatment of VTE, allowing for personalized dosing based on individual risk factors .
Biomarkers and Broader Biological Effects
Recent studies have explored this compound's impact on various biomarkers indicative of biological processes beyond coagulation:
- Reduction in Biomarkers : Clinical studies reported a decrease in D-dimers, thrombin-antithrombin complexes, and prothrombin fragment 1 + 2 following this compound treatment .
- Inflammation and Endothelial Activation : this compound may influence biomarkers related to inflammation (e.g., interleukin-6) and endothelial activation (e.g., matrix metalloproteinase-9), suggesting potential anti-inflammatory effects .
Case Studies
- PREVENT-HD Trial : This trial assessed the efficacy of this compound in preventing thrombotic events in patients with symptomatic COVID-19. While it faced enrollment challenges, it highlighted this compound's safety profile with no critical bleeding events reported .
- Real-world Evidence : Observational studies have corroborated clinical trial findings, showing consistent efficacy and safety profiles across diverse patient populations treated with this compound for VTE .
Q & A
Q. Basic: What standardized protocols ensure analytical validity for rivaroxaban in pharmaceutical formulations?
Methodological Answer:
Analytical validation of this compound requires adherence to guidelines from regulatory authorities (e.g., FDA, ICH, USP). Key parameters include specificity, linearity, accuracy, precision, and robustness. For example, high-performance liquid chromatography (HPLC) methods are validated by assessing recovery rates (95–105%) and repeatability (RSD <2%) using spiked samples. Stability testing under varying pH and temperature conditions is critical to ensure potency and purity .
Q. Basic: How are clinical trial designs optimized to assess this compound’s efficacy in venous thromboembolism (VTE) prophylaxis?
Methodological Answer:
Phase 3 trials like RECORD 1–4 used double-blind, randomized controlled designs with non-inferiority endpoints (e.g., symptomatic VTE incidence). Key considerations include stratification by surgery type (hip/knee replacement) and exclusion of high-bleeding-risk populations. Post-hoc adjustments, such as PT measurements for exposure modeling, address inter-patient variability .
Q. Advanced: How do conflicting efficacy and safety data between this compound and apixaban inform meta-analytical approaches?
Methodological Answer:
Contradictory outcomes (e.g., lower bleeding risk with apixaban in VTE) require pooled hazard ratios with sensitivity analyses to adjust for study heterogeneity. For instance, a 2024 meta-analysis weighted trials by sample size and bleeding definition consistency. Subgroup analyses by renal function and cancer status resolve discrepancies, as apixaban’s safety advantage diminishes in patients with CrCl <50 mL/min .
Q. Advanced: What pharmacokinetic (PK) models predict this compound exposure variability in specific subpopulations?
Methodological Answer:
Population PK models integrate covariates like age, weight, and renal function to simulate exposure (AUC). For example, a two-compartment model with first-order absorption (estimated bioavailability: 80–100%) predicts 40% higher exposure in elderly patients (age >75). Monte Carlo simulations validate these models against observed PT prolongation data, though shallow exposure-response slopes limit clinical utility for dose adjustments .
Q. Advanced: How are dose optimization strategies for this compound evaluated in atrial fibrillation (AF) patients with comorbidities?
Methodological Answer:
The AFIRE Trial used Cox proportional hazards models to compare underdosed (10–15 mg/day) and standard-dose this compound (20 mg/day) in AF patients with stable coronary disease. Despite similar thrombotic event rates, underdosing reduced major bleeding (HR: 0.62; 95% CI: 0.41–0.93). Propensity score matching controlled for renal function and prior stroke, highlighting the need for individualized dosing in high-bleeding-risk cohorts .
Q. Basic: What statistical methods address missing data in this compound persistence studies?
Methodological Answer:
Longitudinal studies (e.g., Dresden Cohort) employ multiple imputation with Markov chain Monte Carlo (MCMC) methods to handle missing follow-up data. Sensitivity analyses (e.g., worst-case scenarios) validate findings, while competing risk models account for mortality and treatment switches. Covariates like heart failure and diabetes are included in Cox models to identify discontinuation predictors .
Q. Advanced: How do pooled analyses resolve conflicting evidence on this compound’s efficacy in high-risk subgroups (e.g., cancer patients)?
Methodological Answer:
Pooled data from EINSTEIN-DVT/PE trials applied inverse variance weighting to compare this compound with enoxaparin/VKA. Interaction tests revealed no efficacy difference in cancer patients (HR: 1.01; 95% CI: 0.74–1.38), but reduced bleeding (HR: 0.56). Stratification by metastasis status and chemotherapy exposure refines risk-benefit assessments .
Q. Basic: What quality control measures ensure consistency in this compound tablet formulations?
Methodological Answer:
Batch testing includes dissolution profiling (USP Apparatus II, 50 rpm, pH 6.8 buffer) and content uniformity (RSD ≤6%). Near-infrared (NIR) spectroscopy monitors blend homogeneity, while HNMR (DMSO-d6 solvent) verifies structural integrity. Regulatory specifications require ±5% deviation from labeled potency .
Q. Advanced: How are machine learning models applied to predict this compound-related adverse events?
Methodological Answer:
Random forest classifiers trained on EHR data (e.g., age, CrCl, concomitant medications) predict major bleeding with 82% accuracy (AUC: 0.79). Feature importance analysis identifies renal impairment and NSAID use as top predictors. External validation in diverse cohorts mitigates overfitting, though model interpretability remains a challenge .
Q. Advanced: What trial designs evaluate this compound’s cost-effectiveness in low-resource settings?
Methodological Answer:
Budget impact analyses (e.g., XAMOS Study) use Markov models with local cost inputs (e.g., enoxaparin hospitalization vs. This compound outpatient care). Incremental cost-effectiveness ratios (ICERs) are calculated for QALYs gained, with sensitivity analyses varying thrombosis recurrence rates (±20%). Probabilistic simulations (10,000 iterations) validate robustness .
Properties
IUPAC Name |
5-chloro-N-[[(5S)-2-oxo-3-[4-(3-oxomorpholin-4-yl)phenyl]-1,3-oxazolidin-5-yl]methyl]thiophene-2-carboxamide | |
---|---|---|
Source | PubChem | |
URL | https://pubchem.ncbi.nlm.nih.gov | |
Description | Data deposited in or computed by PubChem | |
InChI |
InChI=1S/C19H18ClN3O5S/c20-16-6-5-15(29-16)18(25)21-9-14-10-23(19(26)28-14)13-3-1-12(2-4-13)22-7-8-27-11-17(22)24/h1-6,14H,7-11H2,(H,21,25)/t14-/m0/s1 | |
Source | PubChem | |
URL | https://pubchem.ncbi.nlm.nih.gov | |
Description | Data deposited in or computed by PubChem | |
InChI Key |
KGFYHTZWPPHNLQ-AWEZNQCLSA-N | |
Source | PubChem | |
URL | https://pubchem.ncbi.nlm.nih.gov | |
Description | Data deposited in or computed by PubChem | |
Canonical SMILES |
C1COCC(=O)N1C2=CC=C(C=C2)N3CC(OC3=O)CNC(=O)C4=CC=C(S4)Cl | |
Source | PubChem | |
URL | https://pubchem.ncbi.nlm.nih.gov | |
Description | Data deposited in or computed by PubChem | |
Isomeric SMILES |
C1COCC(=O)N1C2=CC=C(C=C2)N3C[C@@H](OC3=O)CNC(=O)C4=CC=C(S4)Cl | |
Source | PubChem | |
URL | https://pubchem.ncbi.nlm.nih.gov | |
Description | Data deposited in or computed by PubChem | |
Molecular Formula |
C19H18ClN3O5S | |
Source | PubChem | |
URL | https://pubchem.ncbi.nlm.nih.gov | |
Description | Data deposited in or computed by PubChem | |
DSSTOX Substance ID |
DTXSID3057723 | |
Record name | Rivaroxaban | |
Source | EPA DSSTox | |
URL | https://comptox.epa.gov/dashboard/DTXSID3057723 | |
Description | DSSTox provides a high quality public chemistry resource for supporting improved predictive toxicology. | |
Molecular Weight |
435.9 g/mol | |
Source | PubChem | |
URL | https://pubchem.ncbi.nlm.nih.gov | |
Description | Data deposited in or computed by PubChem | |
Solubility |
Slightly soluble in organic solvents (e.g. acetone, polyethylene glycol 400) and is pratically insoluble in water and aqueous media | |
Record name | Rivaroxaban | |
Source | Hazardous Substances Data Bank (HSDB) | |
URL | https://pubchem.ncbi.nlm.nih.gov/source/hsdb/8149 | |
Description | The Hazardous Substances Data Bank (HSDB) is a toxicology database that focuses on the toxicology of potentially hazardous chemicals. It provides information on human exposure, industrial hygiene, emergency handling procedures, environmental fate, regulatory requirements, nanomaterials, and related areas. The information in HSDB has been assessed by a Scientific Review Panel. | |
Color/Form |
White to yellowish powder | |
CAS No. |
366789-02-8 | |
Record name | Rivaroxaban | |
Source | CAS Common Chemistry | |
URL | https://commonchemistry.cas.org/detail?cas_rn=366789-02-8 | |
Description | CAS Common Chemistry is an open community resource for accessing chemical information. Nearly 500,000 chemical substances from CAS REGISTRY cover areas of community interest, including common and frequently regulated chemicals, and those relevant to high school and undergraduate chemistry classes. This chemical information, curated by our expert scientists, is provided in alignment with our mission as a division of the American Chemical Society. | |
Explanation | The data from CAS Common Chemistry is provided under a CC-BY-NC 4.0 license, unless otherwise stated. | |
Record name | Rivaroxaban | |
Source | DrugBank | |
URL | https://www.drugbank.ca/drugs/DB06228 | |
Description | The DrugBank database is a unique bioinformatics and cheminformatics resource that combines detailed drug (i.e. chemical, pharmacological and pharmaceutical) data with comprehensive drug target (i.e. sequence, structure, and pathway) information. | |
Explanation | Creative Common's Attribution-NonCommercial 4.0 International License (http://creativecommons.org/licenses/by-nc/4.0/legalcode) | |
Record name | Rivaroxaban | |
Source | EPA DSSTox | |
URL | https://comptox.epa.gov/dashboard/DTXSID3057723 | |
Description | DSSTox provides a high quality public chemistry resource for supporting improved predictive toxicology. | |
Record name | 5-chloro-N-{[(5S)-2-oxo-3-[4-(3-oxomorpholin-4-yl)phenyl]-1,3-oxazolidin-5-yl]methyl}thiophene-2-carboxamide | |
Source | European Chemicals Agency (ECHA) | |
URL | https://echa.europa.eu/information-on-chemicals | |
Description | The European Chemicals Agency (ECHA) is an agency of the European Union which is the driving force among regulatory authorities in implementing the EU's groundbreaking chemicals legislation for the benefit of human health and the environment as well as for innovation and competitiveness. | |
Explanation | Use of the information, documents and data from the ECHA website is subject to the terms and conditions of this Legal Notice, and subject to other binding limitations provided for under applicable law, the information, documents and data made available on the ECHA website may be reproduced, distributed and/or used, totally or in part, for non-commercial purposes provided that ECHA is acknowledged as the source: "Source: European Chemicals Agency, http://echa.europa.eu/". Such acknowledgement must be included in each copy of the material. ECHA permits and encourages organisations and individuals to create links to the ECHA website under the following cumulative conditions: Links can only be made to webpages that provide a link to the Legal Notice page. | |
Record name | RIVAROXABAN | |
Source | FDA Global Substance Registration System (GSRS) | |
URL | https://gsrs.ncats.nih.gov/ginas/app/beta/substances/9NDF7JZ4M3 | |
Description | The FDA Global Substance Registration System (GSRS) enables the efficient and accurate exchange of information on what substances are in regulated products. Instead of relying on names, which vary across regulatory domains, countries, and regions, the GSRS knowledge base makes it possible for substances to be defined by standardized, scientific descriptions. | |
Explanation | Unless otherwise noted, the contents of the FDA website (www.fda.gov), both text and graphics, are not copyrighted. They are in the public domain and may be republished, reprinted and otherwise used freely by anyone without the need to obtain permission from FDA. Credit to the U.S. Food and Drug Administration as the source is appreciated but not required. | |
Record name | Rivaroxaban | |
Source | Hazardous Substances Data Bank (HSDB) | |
URL | https://pubchem.ncbi.nlm.nih.gov/source/hsdb/8149 | |
Description | The Hazardous Substances Data Bank (HSDB) is a toxicology database that focuses on the toxicology of potentially hazardous chemicals. It provides information on human exposure, industrial hygiene, emergency handling procedures, environmental fate, regulatory requirements, nanomaterials, and related areas. The information in HSDB has been assessed by a Scientific Review Panel. | |
Synthesis routes and methods
Procedure details
Retrosynthesis Analysis
AI-Powered Synthesis Planning: Our tool employs the Template_relevance Pistachio, Template_relevance Bkms_metabolic, Template_relevance Pistachio_ringbreaker, Template_relevance Reaxys, Template_relevance Reaxys_biocatalysis model, leveraging a vast database of chemical reactions to predict feasible synthetic routes.
One-Step Synthesis Focus: Specifically designed for one-step synthesis, it provides concise and direct routes for your target compounds, streamlining the synthesis process.
Accurate Predictions: Utilizing the extensive PISTACHIO, BKMS_METABOLIC, PISTACHIO_RINGBREAKER, REAXYS, REAXYS_BIOCATALYSIS database, our tool offers high-accuracy predictions, reflecting the latest in chemical research and data.
Strategy Settings
Precursor scoring | Relevance Heuristic |
---|---|
Min. plausibility | 0.01 |
Model | Template_relevance |
Template Set | Pistachio/Bkms_metabolic/Pistachio_ringbreaker/Reaxys/Reaxys_biocatalysis |
Top-N result to add to graph | 6 |
Feasible Synthetic Routes
Disclaimer and Information on In-Vitro Research Products
Please be aware that all articles and product information presented on BenchChem are intended solely for informational purposes. The products available for purchase on BenchChem are specifically designed for in-vitro studies, which are conducted outside of living organisms. In-vitro studies, derived from the Latin term "in glass," involve experiments performed in controlled laboratory settings using cells or tissues. It is important to note that these products are not categorized as medicines or drugs, and they have not received approval from the FDA for the prevention, treatment, or cure of any medical condition, ailment, or disease. We must emphasize that any form of bodily introduction of these products into humans or animals is strictly prohibited by law. It is essential to adhere to these guidelines to ensure compliance with legal and ethical standards in research and experimentation.