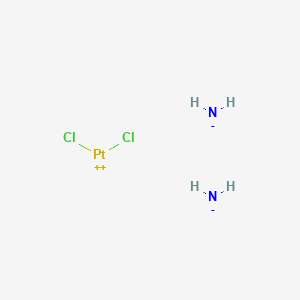
Azanide; dichloroplatinum(2+)
- Click on QUICK INQUIRY to receive a quote from our team of experts.
- With the quality product at a COMPETITIVE price, you can focus more on your research.
Overview
Description
Mechanism of Action
Target of Action
Cisplatin, a platinum-based chemotherapy agent, primarily targets DNA within the cell . It forms covalent bonds with the purine bases of DNA, leading to the formation of intra- and inter-strand DNA adducts .
Mode of Action
Cisplatin interacts with DNA to form cisplatin-DNA adducts, which cause DNA damage and inhibit DNA synthesis . This interaction leads to cell cycle arrest and triggers apoptosis . The cisplatin-modified DNA is poorly repaired due to a shielding effect caused by proteins attracted to the modified DNA .
Biochemical Pathways
Cisplatin affects several biochemical pathways. It activates three major pathways of apoptosis: the extrinsic pathway mediated by death receptors, the intrinsic pathway centered on mitochondria, and the endoplasmic reticulum (ER)-stress pathway . The drug induces its cytotoxic properties through binding to nuclear DNA and subsequent interference with normal transcription and/or DNA replication mechanisms .
Pharmacokinetics
The pharmacokinetics of cisplatin are complex . It is absorbed into the cancer cell and interacts with cellular macromolecules . The pharmacokinetics of cisplatin can be influenced by patient characteristics .
Result of Action
The primary result of cisplatin’s action is the induction of cell death through apoptosis . The drug’s interaction with DNA leads to DNA damage, cell cycle arrest, and ultimately, apoptosis . Cisplatin also induces both intrinsic and extrinsic pathways of apoptosis, resulting in the production of reactive oxygen species, activation of various signal transduction pathways, upregulation of pro-apoptotic genes/proteins, and down-regulation of proto-oncogenes and anti-apoptotic genes/proteins .
Action Environment
The action of cisplatin can be influenced by the cellular environment. For instance, the drug’s diffusion can be dependent on membrane composition . Additionally, the drug’s effectiveness can be hindered by the development of resistance, leading to cancer relapse . Various mechanisms contribute to cisplatin resistance, including diminished intracellular cisplatin accumulation, intracellular detoxification, DNA repair, autophagy responses, heat shock proteins, tumor microenvironment, cancer stem cells, epigenetic regulation, ferroptosis resistance, and metabolic reprogramming .
Biochemical Analysis
Biochemical Properties
Cisplatin plays a significant role in biochemical reactions. It interacts with various enzymes, proteins, and other biomolecules. The copper transporter 1 is particularly important for cisplatin uptake in tumor cells . Cisplatin also interacts with the organic cation transporter 2, which may play a major role in the development of undesired cisplatin side effects .
Cellular Effects
Cisplatin has profound effects on various types of cells and cellular processes. It influences cell function by interfering with transcription and/or DNA replication mechanisms . It also induces cytotoxicity, depending on cell type and concentration . Cisplatin damages tumors via induction of apoptosis, mediated by the activation of various signal transduction pathways .
Molecular Mechanism
Cisplatin exerts its effects at the molecular level through a series of steps: cellular uptake, activation by aquation, DNA binding, and the processing of DNA lesions . These steps may alter both DNA transcription and RNA replication, leading to cancer cell death .
Temporal Effects in Laboratory Settings
In laboratory settings, the effects of cisplatin change over time. For instance, cisplatin induces multiple episodes of acute kidney injury (AKI), leading to chronic kidney disease (CKD) after the 4th weekly dose . This suggests that cisplatin has long-term effects on cellular function observed in in vitro or in vivo studies .
Dosage Effects in Animal Models
The effects of cisplatin vary with different dosages in animal models. Higher doses of cisplatin result in higher cisplatin tissue retention . Importantly, cisplatin protocol, hydration, and supportive care all together affect not only the maximum tolerated dose or lethal dose but also the therapeutic dose of cisplatin and its side effects .
Metabolic Pathways
Cisplatin is involved in various metabolic pathways. It interacts with enzymes or cofactors and can affect metabolic flux or metabolite levels . Metabolic pathways show strong connections between cisplatin resistance and metabolic reprogramming including glycolysis, glutamine metabolism, and fatty acid metabolism .
Transport and Distribution
Cisplatin is transported and distributed within cells and tissues. It interacts with transporters such as ATP7A and ATP7B, which mediate its efflux from the cell or its distribution to specific sub-cellular compartments .
Subcellular Localization
Cisplatin is extensively sequestered into vesicular structures of the lysosomal, Golgi, and secretory compartments . The detection of cisplatin in the vesicles of the secretory pathway is consistent with a major role for this pathway in the efflux of cisplatin from the cell .
Preparation Methods
Azanide; dichloroplatinum(2+) is synthesized through a series of chemical reactions involving platinum compounds. The most common synthetic route involves the reaction of potassium tetrachloroplatinate(II) with ammonia to form cis-diamminedichloroplatinum(II). The reaction conditions typically include an aqueous medium and controlled temperature to ensure the formation of the desired cis isomer . Industrial production methods often involve large-scale synthesis in controlled environments to maintain the purity and efficacy of the compound .
Chemical Reactions Analysis
Azanide; dichloroplatinum(2+) undergoes several types of chemical reactions, including hydrolysis, substitution, and reduction. One of the key reactions is hydrolysis, where the chloride ligands are replaced by water molecules, forming aquated species such as cis-[PtCl(NH3)2(H2O)]+ . These aquated species are highly reactive and can further react with nucleophiles, leading to the formation of DNA adducts, which are crucial for its anticancer activity . Common reagents used in these reactions include water, ammonia, and various nucleophiles . The major products formed from these reactions are DNA adducts and other platinum complexes .
Scientific Research Applications
Azanide; dichloroplatinum(2+) has a wide range of scientific research applications, particularly in the fields of chemistry, biology, medicine, and industry. In chemistry, it is used as a model compound to study the coordination chemistry of platinum complexes . In biology, cisplatin is used to investigate the mechanisms of DNA damage and repair . In medicine, it is a critical component of chemotherapy regimens for various cancers . Additionally, cisplatin is used in industrial applications, such as the development of new drug delivery systems and the study of drug resistance mechanisms .
Comparison with Similar Compounds
Azanide; dichloroplatinum(2+) is part of a class of platinum-based chemotherapy drugs, which also includes carboplatin and oxaliplatin . While all three compounds share a similar mechanism of action, they differ in their chemical structures and side effect profiles . Carboplatin, for example, has a bidentate dicarboxylate ligand instead of the chloride ligands found in cisplatin, which results in a different toxicity profile and reduced side effects . Oxaliplatin, on the other hand, has a diaminocyclohexane ligand, which makes it effective against some cisplatin-resistant cancers . Other similar compounds include nedaplatin, lobaplatin, and heptaplatin, each with unique properties and clinical applications .
Properties
Alkylating agents work by three different mechanisms: 1) attachment of alkyl groups to DNA bases, resulting in the DNA being fragmented by repair enzymes in their attempts to replace the alkylated bases, preventing DNA synthesis and RNA transcription from the affected DNA, 2) DNA damage via the formation of cross-links (bonds between atoms in the DNA) which prevents DNA from being separated for synthesis or transcription, and 3) the induction of mispairing of the nucleotides leading to mutations. | |
CAS No. |
15663-27-1 |
Molecular Formula |
Cl2H6N2Pt |
Molecular Weight |
300.05 g/mol |
IUPAC Name |
azane;dichloroplatinum |
InChI |
InChI=1S/2ClH.2H3N.Pt/h2*1H;2*1H3;/q;;;;+2/p-2 |
InChI Key |
LXZZYRPGZAFOLE-UHFFFAOYSA-L |
SMILES |
[NH2-].[NH2-].Cl[Pt+2]Cl |
Canonical SMILES |
N.N.Cl[Pt]Cl |
Appearance |
Yellow solid powder |
melting_point |
270 dec °C |
14283-03-5 | |
physical_description |
Yellow crystalline solid; [MSDSonline] |
Pictograms |
Corrosive; Irritant; Health Hazard |
Purity |
>98% (or refer to the Certificate of Analysis) |
shelf_life |
>2 years if stored properly |
solubility |
H2O 1 (mg/mL) DMSO 10 (mg/mL) 10:1 PVP coprecipitate in 10% PVP 2.5 (mg/mL) Dimethylformamide (pure anhydrous) 24 (mg/mL) |
storage |
Dry, dark and at 0 - 4 C for short term (days to weeks) or -20 C for long term (months to years). |
Synonyms |
CACP; cisDDP; cisdiamminedichloro platinum (II); cisdiamminedichloroplatinum; Cisdichloroammine Platinum (II); Cismaplat; Cisplatina; cisplatinous diamine dichloride; cisplatinum; cisplatinum II; cisplatinum II diamine dichloride; CPDD; Cysplatyna; DDP; PDD; Peyrones Chloride; Peyrones Salt; Platinoxan; platinum diamminodichloride; US brand names: Platinol; PlatinolAQ; Foreign brand names: Abiplatin; Blastolem; Briplatin; Cisplatyl; Citoplatino; Citosin; Lederplatin; Metaplatin; Neoplatin; Placis; Platamine; Platiblastin; PlatiblastinS; Platinex; Platinol AQ; PlatinolAQ VHA Plus; Platiran; Platistin; Platosin; Abbreviations: CDDP; DDP. |
Origin of Product |
United States |
Disclaimer and Information on In-Vitro Research Products
Please be aware that all articles and product information presented on BenchChem are intended solely for informational purposes. The products available for purchase on BenchChem are specifically designed for in-vitro studies, which are conducted outside of living organisms. In-vitro studies, derived from the Latin term "in glass," involve experiments performed in controlled laboratory settings using cells or tissues. It is important to note that these products are not categorized as medicines or drugs, and they have not received approval from the FDA for the prevention, treatment, or cure of any medical condition, ailment, or disease. We must emphasize that any form of bodily introduction of these products into humans or animals is strictly prohibited by law. It is essential to adhere to these guidelines to ensure compliance with legal and ethical standards in research and experimentation.