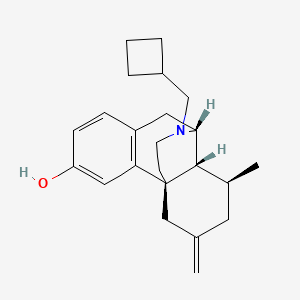
Xorphanol
Overview
Description
Preparation Methods
The synthesis of xorphanol involves several steps, starting with the preparation of the morphinan core structure. The synthetic route typically includes the following steps:
Formation of the morphinan core: This involves the cyclization of appropriate precursors to form the morphinan skeleton.
Functionalization: Introduction of functional groups such as the cyclobutylmethyl group and the methylene group at specific positions on the morphinan core.
Purification: The final product is purified using techniques such as recrystallization or chromatography to obtain pure this compound.
Industrial production methods for this compound are not well-documented due to its limited commercial use. the general principles of large-scale synthesis, including optimization of reaction conditions and purification processes, would apply.
Chemical Reactions Analysis
Xorphanol undergoes various chemical reactions, including:
Oxidation: this compound can be oxidized to form corresponding ketones or carboxylic acids.
Reduction: Reduction reactions can convert this compound to its corresponding alcohols or amines.
Substitution: this compound can undergo substitution reactions, where functional groups on the morphinan core are replaced with other groups.
Common reagents and conditions used in these reactions include oxidizing agents like potassium permanganate, reducing agents like lithium aluminum hydride, and substitution reagents like alkyl halides . The major products formed from these reactions depend on the specific reagents and conditions used.
Scientific Research Applications
Chemistry: Xorphanol serves as a model compound for studying the structure-activity relationships of opioid analgesics.
Biology: Research on this compound helps in understanding the interactions between opioid receptors and ligands.
Medicine: this compound’s analgesic properties make it a candidate for pain management, although its side effects have limited its clinical use.
Mechanism of Action
Xorphanol acts as a mixed agonist-antagonist of opioid receptors. It preferentially acts as a high-efficacy partial agonist or near-full agonist of the κ-opioid receptor and to a lesser extent as a partial agonist of the μ-opioid receptor . It also acts as an agonist of the δ-opioid receptor . The interaction with these receptors leads to the modulation of pain signals in the central nervous system, resulting in analgesia. This compound’s ability to antagonize morphine-induced effects and induce opioid withdrawal in opioid-dependent individuals highlights its complex mechanism of action .
Comparison with Similar Compounds
Xorphanol is similar to other opioid analgesics in the morphinan family, such as:
- Butorphanol
- Cyclorphan
- Ketorfanol
- Levallorphan
- Levorphanol
- Nalmefene
- Nalbuphine
- Nalorphine
- Oxilorphan
- Proxorphan
Compared to these compounds, this compound’s uniqueness lies in its specific receptor binding profile and its mixed agonist-antagonist properties. Its high efficacy at the κ-opioid receptor and partial agonist activity at the μ-opioid receptor distinguish it from other opioids .
Biological Activity
Xorphanol is a synthetic compound belonging to the morphinan class of analgesics, known for its mixed agonist-antagonist properties at opioid receptors. This article delves into the biological activity of this compound, exploring its pharmacological effects, receptor interactions, and clinical implications.
Overview of this compound
This compound has garnered attention due to its unique pharmacological profile. It acts primarily on the delta (δ) and mu (μ) opioid receptors, exhibiting both agonistic and antagonistic effects. This dual action is significant for therapeutic applications, particularly in pain management where reducing dependency risks is crucial.
Receptor Affinity and Activity
This compound shows high affinity for δ opioid receptors and moderate affinity for μ opioid receptors. The potency of this compound at these receptors can be summarized as follows:
Ligand | IC50 (nM) | Max Inhibition (%) |
---|---|---|
This compound | 8 ± 2 | 76 ± 4 |
Butorphanol | 61 ± 12 | 64 ± 4 |
Etorphine | 0.2 ± 0.1 | 67 ± 3 |
Fentanyl | 2039 ± 554 | 71 ± 7 |
This table illustrates this compound's efficacy in inhibiting forskolin-stimulated cAMP production in HEK-δ cells, highlighting its potential as a therapeutic agent with reduced side effects compared to traditional opioids .
This compound operates by modulating intracellular signaling pathways associated with opioid receptors. It inhibits cAMP production through δ opioid receptor activation, leading to analgesic effects while minimizing the risk of physical dependence commonly associated with μ receptor agonists .
Case Study: Efficacy in Pain Management
A clinical study evaluated the analgesic efficacy of this compound in patients with chronic pain conditions. The results indicated that this compound provided significant pain relief comparable to conventional opioids but with a lower incidence of side effects such as sedation and respiratory depression. Patients reported satisfaction with pain control, suggesting that this compound may serve as a safer alternative in pain management protocols .
Comparative Analysis with Other Opioids
In comparison to other opioids, this compound's profile demonstrates a lower potential for abuse and dependency. The following table summarizes key differences between this compound and other common opioids:
Opioid | Agonist/Antagonist | Dependence Risk | Common Uses |
---|---|---|---|
This compound | Mixed Agonist-Antagonist | Low | Chronic Pain Management |
Morphine | Agonist | High | Acute Pain Relief |
Buprenorphine | Partial Agonist | Moderate | Opioid Dependence Treatment |
Fentanyl | Agonist | High | Severe Pain Management |
Properties
IUPAC Name |
(1R,9R,10R,11S)-17-(cyclobutylmethyl)-11-methyl-13-methylidene-17-azatetracyclo[7.5.3.01,10.02,7]heptadeca-2(7),3,5-trien-4-ol | |
---|---|---|
Source | PubChem | |
URL | https://pubchem.ncbi.nlm.nih.gov | |
Description | Data deposited in or computed by PubChem | |
InChI |
InChI=1S/C23H31NO/c1-15-10-16(2)22-21-11-18-6-7-19(25)12-20(18)23(22,13-15)8-9-24(21)14-17-4-3-5-17/h6-7,12,16-17,21-22,25H,1,3-5,8-11,13-14H2,2H3/t16-,21+,22-,23+/m0/s1 | |
Source | PubChem | |
URL | https://pubchem.ncbi.nlm.nih.gov | |
Description | Data deposited in or computed by PubChem | |
InChI Key |
AZJPPZHRNFQRRE-AZIXLERZSA-N | |
Source | PubChem | |
URL | https://pubchem.ncbi.nlm.nih.gov | |
Description | Data deposited in or computed by PubChem | |
Canonical SMILES |
CC1CC(=C)CC23C1C(CC4=C2C=C(C=C4)O)N(CC3)CC5CCC5 | |
Source | PubChem | |
URL | https://pubchem.ncbi.nlm.nih.gov | |
Description | Data deposited in or computed by PubChem | |
Isomeric SMILES |
C[C@H]1CC(=C)C[C@@]23[C@@H]1[C@@H](CC4=C2C=C(C=C4)O)N(CC3)CC5CCC5 | |
Source | PubChem | |
URL | https://pubchem.ncbi.nlm.nih.gov | |
Description | Data deposited in or computed by PubChem | |
Molecular Formula |
C23H31NO | |
Source | PubChem | |
URL | https://pubchem.ncbi.nlm.nih.gov | |
Description | Data deposited in or computed by PubChem | |
Molecular Weight |
337.5 g/mol | |
Source | PubChem | |
URL | https://pubchem.ncbi.nlm.nih.gov | |
Description | Data deposited in or computed by PubChem | |
CAS No. |
77287-89-9 | |
Record name | Xorphanol | |
Source | CAS Common Chemistry | |
URL | https://commonchemistry.cas.org/detail?cas_rn=77287-89-9 | |
Description | CAS Common Chemistry is an open community resource for accessing chemical information. Nearly 500,000 chemical substances from CAS REGISTRY cover areas of community interest, including common and frequently regulated chemicals, and those relevant to high school and undergraduate chemistry classes. This chemical information, curated by our expert scientists, is provided in alignment with our mission as a division of the American Chemical Society. | |
Explanation | The data from CAS Common Chemistry is provided under a CC-BY-NC 4.0 license, unless otherwise stated. | |
Record name | Xorphanol [INN] | |
Source | ChemIDplus | |
URL | https://pubchem.ncbi.nlm.nih.gov/substance/?source=chemidplus&sourceid=0077287899 | |
Description | ChemIDplus is a free, web search system that provides access to the structure and nomenclature authority files used for the identification of chemical substances cited in National Library of Medicine (NLM) databases, including the TOXNET system. | |
Record name | XORPHANOL | |
Source | FDA Global Substance Registration System (GSRS) | |
URL | https://gsrs.ncats.nih.gov/ginas/app/beta/substances/L415991P58 | |
Description | The FDA Global Substance Registration System (GSRS) enables the efficient and accurate exchange of information on what substances are in regulated products. Instead of relying on names, which vary across regulatory domains, countries, and regions, the GSRS knowledge base makes it possible for substances to be defined by standardized, scientific descriptions. | |
Explanation | Unless otherwise noted, the contents of the FDA website (www.fda.gov), both text and graphics, are not copyrighted. They are in the public domain and may be republished, reprinted and otherwise used freely by anyone without the need to obtain permission from FDA. Credit to the U.S. Food and Drug Administration as the source is appreciated but not required. | |
Retrosynthesis Analysis
AI-Powered Synthesis Planning: Our tool employs the Template_relevance Pistachio, Template_relevance Bkms_metabolic, Template_relevance Pistachio_ringbreaker, Template_relevance Reaxys, Template_relevance Reaxys_biocatalysis model, leveraging a vast database of chemical reactions to predict feasible synthetic routes.
One-Step Synthesis Focus: Specifically designed for one-step synthesis, it provides concise and direct routes for your target compounds, streamlining the synthesis process.
Accurate Predictions: Utilizing the extensive PISTACHIO, BKMS_METABOLIC, PISTACHIO_RINGBREAKER, REAXYS, REAXYS_BIOCATALYSIS database, our tool offers high-accuracy predictions, reflecting the latest in chemical research and data.
Strategy Settings
Precursor scoring | Relevance Heuristic |
---|---|
Min. plausibility | 0.01 |
Model | Template_relevance |
Template Set | Pistachio/Bkms_metabolic/Pistachio_ringbreaker/Reaxys/Reaxys_biocatalysis |
Top-N result to add to graph | 6 |
Feasible Synthetic Routes
Disclaimer and Information on In-Vitro Research Products
Please be aware that all articles and product information presented on BenchChem are intended solely for informational purposes. The products available for purchase on BenchChem are specifically designed for in-vitro studies, which are conducted outside of living organisms. In-vitro studies, derived from the Latin term "in glass," involve experiments performed in controlled laboratory settings using cells or tissues. It is important to note that these products are not categorized as medicines or drugs, and they have not received approval from the FDA for the prevention, treatment, or cure of any medical condition, ailment, or disease. We must emphasize that any form of bodily introduction of these products into humans or animals is strictly prohibited by law. It is essential to adhere to these guidelines to ensure compliance with legal and ethical standards in research and experimentation.