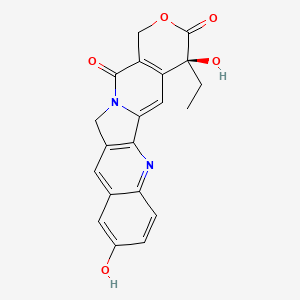
10-Hydroxycamptothecin
Overview
Description
10-Hydroxycamptothecin (10-HCPT) is a natural indole alkaloid derived from Camptotheca acuminata and a metabolite of camptothecin (CPT). It inhibits topoisomerase I (Topo I) by stabilizing the Topo I-DNA complex, thereby blocking DNA replication and inducing apoptosis . Compared to CPT, 10-HCPT exhibits enhanced antitumor efficacy, reduced toxicity, and improved metabolic stability, making it a clinically relevant chemotherapeutic agent . Its molecular formula is C20H16N2O5, with a molecular weight of 364.36 g/mol .
Preparation Methods
Chemical Synthesis Approaches
Platinum-Catalyzed Hydrogenation of Camptothecin
The foundational method for synthesizing 10-hydroxycamptothecin involves the catalytic hydrogenation of camptothecin using platinum-based catalysts. In this process, camptothecin (3.2 g, 0.0092 mol) is dissolved in acetic acid and subjected to hydrogen gas (1 atm) in the presence of pre-reduced platinum oxide (0.8 g) for 8.5 hours. The reaction proceeds via selective hydrogenation of the camptothecin lactone ring, yielding a mixture of 1,2,6,7-tetrahydroxycamptothecin intermediates. Subsequent oxidation with lead(IV) acetate (6.4 g, 0.014 mol) facilitates aromatization, producing this compound as the major product (44% yield). Challenges in this method include incomplete conversion, necessitating purification via high-performance liquid chromatography (HPLC) to isolate this compound from unreacted camptothecin (32%) and acetylated byproducts (26%).
Table 1: Reaction Conditions and Yields for Platinum-Catalyzed Synthesis
Parameter | Value |
---|---|
Catalyst | Platinum oxide (PtO₂) |
Reaction Time | 8.5 hours |
Hydrogen Pressure | 1 atm |
Oxidation Agent | Lead(IV) acetate |
Crude Yield | 44% this compound |
Byproducts | 26% Acetylated derivatives |
Acid-Base Microprecipitation for Nanocrystal Synthesis
To address solubility limitations, researchers have developed stabilizer-free nanocrystals using acid-base microprecipitation. In this method, this compound powder (25 mg) is dissolved in 0.1 M sodium hydroxide (2 mL) and rapidly acidified with 0.1 M hydrochloric acid (2 mL) under sonication. The pH-dependent conversion of the carboxylate form to the lactone form induces precipitation, yielding nanocrystals with a mean particle size of 130 nm and polydispersity index (PDI) of 0.13. High-pressure homogenization (2,000 bar, 8 cycles) further refines particle size distribution, achieving a drug-loading content of 75%. This approach eliminates organic solvents, enhancing biocompatibility for intravenous administration.
Prodrug Derivatization Strategies
Amino Acid-Conjugated Prodrugs
Prodrug design aims to improve this compound’s pharmacokinetic profile. A notable strategy involves conjugating the compound with amino acids via isocyanate intermediates. For example, reacting this compound with glycine benzyl ester in the presence of bis(trichloromethyl) carbonate yields a water-soluble prodrug. Catalytic hydrogenation removes the benzyl protecting group, releasing the active drug in vivo. These prodrugs exhibit >35% conversion within 1 hour in physiological conditions, with complete release achieved after 24 hours.
Table 2: Prodrug Conversion Rates Under Physiological Conditions
Prodrug Structure | Conversion Rate (1 hour) | Complete Conversion Time |
---|---|---|
Glycine conjugate | 38% | 24 hours |
Phenylalanine conjugate | 42% | 18 hours |
Norcantharidin Hybrid Conjugates
Hybrid conjugates of this compound and norcantharidin (a protein phosphatase inhibitor) demonstrate synergistic anticancer effects. Using (3-dimethylaminopropyl)ethyl-carbodiimide monohydrochloride (EDCI) as a coupling agent, researchers synthesized 10-phenolic esters with norcantharidin monoacid derivatives. These conjugates suppress cancer cell growth in vitro at IC₅₀ values of 1.2–3.8 μM, outperforming parent compounds in apoptosis induction.
Nanocrystalline Formulations for Enhanced Delivery
Antisolvent Precipitation Combined with High-Pressure Homogenization
A two-step method involving antisolvent precipitation (AP) and high-pressure homogenization (HPH) produces nanocolloidal particles with improved solubility. Dimethyl sulfoxide (DMSO) serves as the solvent, with water as the antisolvent at a 1:5 volume ratio. HPH at 15,000 psi for 10 cycles reduces particle size to 85 nm, while poloxamer 188 (0.5% w/v) stabilizes the dispersion. Lyophilization with mannitol (2:1 drug-to-lyoprotectant ratio) yields a shelf-stable powder with 98% encapsulation efficiency.
Table 3: Optimization Parameters for Nanocrystal Preparation
Parameter | Optimal Value | Effect on Particle Size |
---|---|---|
DMSO:H₂O Ratio | 1:5 | Minimizes aggregation |
Homogenization Pressure | 15,000 psi | Reduces size to 85 nm |
Poloxamer 188 Conc. | 0.5% w/v | Prevents Ostwald ripening |
Stabilizer-Free Nanocrystals via pH Modulation
Eliminating stabilizers, a pH-modulated method dissolves this compound in alkaline solution (pH 12) and titrates to pH 5 using hydrochloric acid. The lactone form precipitates as nanocrystals, which are homogenized (2,000 bar, 8 cycles) and lyophilized. This formulation achieves 75% drug loading and sustains release over 72 hours in vitro, making it suitable for depot injections.
Analytical Characterization Techniques
High-Performance Liquid Chromatography (HPLC)
Reverse-phase HPLC with C18 columns remains the gold standard for quantifying this compound. Using a mobile phase of acetonitrile:water (45:55, 0.1% trifluoroacetic acid), analysts achieve baseline separation of this compound from camptothecin and acetylated derivatives in 12 minutes. UV detection at 254 nm provides a linear response (R² = 0.999) across 10–1,000 ng/mL, with a limit of detection (LOD) of 2.62 ng/mL.
Solid-Phase Microextraction (SPME) for Plasma Analysis
Biocompatible SPME fibers coated with poly(methacrylic acid-ethylene glycol dimethacrylate) extract this compound directly from human plasma. Coupled with HPLC-fluorescence detection, this method attains an LOD of 0.1 ng/mL, enabling pharmacokinetic studies at subtherapeutic doses.
Chemical Reactions Analysis
Types of Reactions: (S)-10-Hydroxycamptothecin undergoes various chemical reactions, including:
Oxidation: Converts the compound into different derivatives with potential therapeutic applications.
Reduction: Alters the functional groups, potentially modifying its biological activity.
Substitution: Involves replacing specific atoms or groups within the molecule to create new compounds with varied properties.
Common Reagents and Conditions:
Oxidation: Reagents like diacetoxyiodobenzene and hydrogen peroxide are commonly used.
Reduction: Catalysts such as palladium on carbon (Pd/C) are employed.
Substitution: Various halogenating agents and nucleophiles are utilized under controlled conditions.
Major Products: The major products formed from these reactions include various hydroxycamptothecin derivatives, each with unique biological activities and potential therapeutic uses .
Scientific Research Applications
(S)-10-Hydroxycamptothecin has a wide range of applications in scientific research:
Chemistry: Used as a model compound to study the mechanisms of DNA topoisomerase I inhibition.
Biology: Investigated for its effects on cell cycle regulation and apoptosis in cancer cells.
Industry: Utilized in the development of new pharmaceuticals and therapeutic agents.
Mechanism of Action
(S)-10-Hydroxycamptothecin exerts its effects primarily by inhibiting DNA topoisomerase I. This enzyme is responsible for relieving torsional strain in DNA during replication and transcription. By stabilizing the enzyme-DNA complex, (S)-10-Hydroxycamptothecin prevents the re-ligation of DNA strands, leading to DNA damage and apoptosis in rapidly dividing cancer cells . The molecular targets include the DNA topoisomerase I enzyme and the DNA itself, disrupting essential cellular processes.
Comparison with Similar Compounds
Camptothecin (CPT)
- Mechanism & Activity : Both CPT and 10-HCPT target Topo I, but 10-HCPT forms more stable DNA complexes, leading to prolonged cell cycle arrest and apoptosis .
- Toxicity: 10-HCPT demonstrates lower systemic toxicity, attributed to its reduced non-specific binding to plasma proteins compared to CPT .
- Stability : The lactone ring of CPT is prone to hydrolysis at physiological pH, reducing its active form. In contrast, 10-HCPT retains 60–90% lactone stability under similar conditions, enhancing bioavailability .
- Formulations: CPT’s poor solubility limits clinical use, whereas 10-HCPT has been successfully formulated in liposomes, cyclodextrin complexes, and PEGylated nanoparticles to improve solubility and tumor targeting .
Irinotecan and Topotecan
- Structural Basis: Irinotecan (prodrug of SN-38) and topotecan are semi-synthetic derivatives of CPT, modified for water solubility. 10-HCPT lacks these synthetic modifications but shares a hydroxy group at position 10, critical for Topo I inhibition .
- Efficacy: In HCT116 and A549 cancer cells, 10-HCPT exhibits comparable cytotoxicity to irinotecan and topotecan at IC50 values of 0.5–1.2 μM .
- Clinical Use: While irinotecan and topotecan are FDA-approved for colorectal and ovarian cancers, 10-HCPT is predominantly used in China for esophageal and colon cancers .
9-Methoxycamptothecin (9-MCPT)
- Source & Activity : Both 9-MCPT and 10-HCPT are naturally occurring CPT derivatives isolated from Camptotheca acuminata and endophytic fungi. They exhibit potent cytotoxicity, with 10-HCPT showing superior Topo I inhibition in vitro (IC50 0.8 μM vs. 1.5 μM for 9-MCPT) .
- Toxicity Profile : 9-MCPT and 10-HCPT lack the severe hemorrhagic cystitis associated with CPT, but 10-HCPT has a broader therapeutic window in vivo .
Novel Derivatives (e.g., Compound 159)
- Enhanced Stability : Compound 159, a synthetic CPT derivative, retains 90% lactone stability in PBS (vs. 30% for CPT and 60% for 10-HCPT), improving pharmacokinetics .
- Cytotoxicity : In HCT116 cells, compound 159 showed IC50 values of 0.3 μM, outperforming 10-HCPT (0.5 μM) and CPT (1.0 μM) .
Key Research Findings
Inhibition Rates in SiHa Cells
A study comparing 10-HCPT with Taxol, Epirubicin, and Oxaliplatin reported:
- At 10 μM, 10-HCPT achieved 72% inhibition of SiHa cells (vs. 68% for Taxol and 65% for Epirubicin), though a novel compound (LG003) showed superior efficacy (85% inhibition, p < 0.01) .
Formulation Advances
- Cyclodextrin-Liposome Systems : 10-HCPT-loaded cyclodextrin-liposome hybrids increased solubility by 12-fold and inhibited HepG-2, A549, and SGC-7901 cells more effectively than free 10-HCPT (p < 0.05) .
- PEG Conjugates: Water-soluble PEG-10-HCPT conjugates reduced toxicity in mice while maintaining antitumor efficacy in xenograft models .
Synergistic Therapies
- Photothermal-Chemotherapy: Hyaluronic acid-modified Prussian blue nanoparticles loaded with 10-HCPT enhanced tumor suppression in vivo by 40% compared to monotherapy (p < 0.01) .
Biological Activity
10-Hydroxycamptothecin (HCPT) is a potent derivative of camptothecin, primarily known for its significant antitumor activity. This compound exerts its therapeutic effects mainly through the inhibition of topoisomerase I (TOP I), an essential enzyme involved in DNA replication and transcription. This article reviews the biological activity of HCPT, focusing on its mechanisms, efficacy in various cancers, and advancements in drug delivery systems.
HCPT's primary mechanism involves the stabilization of the TOP I-DNA cleavable complex, leading to DNA damage and subsequent apoptosis in cancer cells. The inhibition of TOP I disrupts the normal progression of the cell cycle, particularly affecting the G2/M phase transition.
Key Findings:
- Cell Cycle Arrest : HCPT treatment results in a significant reduction in cyclin B1 levels and an increase in p21 expression, leading to G2/M phase arrest in various cancer cell lines, including esophageal squamous cell carcinoma (ESCC) .
- Induction of Apoptosis : The compound promotes apoptosis as evidenced by increased levels of cleaved PARP, caspases-3 and -7, and pro-apoptotic proteins such as Bax and Bim, alongside a decrease in anti-apoptotic Bcl-2 .
Efficacy Against Different Cancers
HCPT has demonstrated potent antitumor effects across various cancer types:
- Esophageal Squamous Cell Carcinoma (ESCC) : In vitro studies showed that HCPT significantly inhibited cell proliferation and induced apoptosis in ESCC cells. In vivo studies further confirmed its efficacy by reducing tumor growth in xenograft models .
- Liver Cancer : HCPT nanosuspensions exhibited higher cytotoxicity against HepG2 cells compared to traditional formulations, with a lower IC50 value indicating enhanced effectiveness .
- Non-Small Cell Lung Cancer (NSCLC) : A combination therapy involving HCPT and crizotinib showed synergistic effects on EGFR- and KRAS-mutant lung cancer cells, enhancing apoptosis without increasing toxicity to normal cells .
Advances in Drug Delivery Systems
Recent advancements in nanotechnology have improved the therapeutic potential of HCPT by enhancing its solubility and stability:
These formulations have shown significant improvements over conventional HCPT injections, suggesting a promising direction for future clinical applications.
Case Studies
- Case Study on ESCC : A study demonstrated that treatment with HCPT led to a substantial decrease in tumor size and proliferation markers (Ki-67) while increasing apoptotic markers (cleaved caspases) in ESCC xenografts .
- Combination Therapy with Crizotinib : In NSCLC models, the combination of HCPT with crizotinib resulted in enhanced inhibition of cell growth and increased apoptosis through mitochondrial signaling pathways .
Q & A
Basic Research Questions
Q. What experimental methodologies are most effective for evaluating 10-HCPT’s inhibition of DNA topoisomerase I in vitro?
- Methodological Answer : Use plasmid DNA cleavage assays to quantify 10-HCPT’s ability to stabilize DNA-topoisomerase I complexes. For example, incubate pBR322 plasmid DNA with recombinant human topoisomerase I and varying concentrations of 10-HCPT. Resolve cleavage products via agarose gel electrophoresis and calculate EC50 values (e.g., EC50 = 0.35 µM for 10-HCPT vs. 18.85 µM for camptothecin) . Pair this with γH2A.X immunofluorescence staining (e.g., ab2893 antibody) to confirm DNA damage in treated cells (e.g., HeLa cells) .
Q. How should researchers design dose-response studies to assess 10-HCPT’s cytotoxicity across cancer cell lines?
- Methodological Answer : Conduct MTT assays on diverse cell lines (e.g., BT-20, MDA-231, Colo 205) exposed to 10-HCPT for 72 hours. Report IC50 values (e.g., 7.27 nM in MDA-231 vs. 34.3 nM in BT-20) and correlate results with cell cycle analysis (flow cytometry) to identify G2/M arrest and caspase-3-dependent apoptosis . Include positive controls (e.g., camptothecin) to benchmark potency differences.
Q. What in vitro models best replicate 10-HCPT’s anti-angiogenic effects observed in vivo?
- Methodological Answer : Use human microvascular endothelial cells (HMECs) to model angiogenesis. Assess migration via scratch assays (IC50 = 0.63 µM) and tube formation via Matrigel-based assays (IC50 = 0.96 µM). Validate findings with CAM (chick chorioallantoic membrane) models, where 25 nM 10-HCPT inhibits 95% of vascular growth .
Q. How can researchers ensure reproducibility in pharmacokinetic studies of 10-HCPT in murine models?
- Methodological Answer : Administer 10-HCPT orally (2.5–7.5 mg/kg every 48 hours) to Colo 205 xenograft mice. Monitor tumor volume and serum concentrations via HPLC-MS. Include toxicity endpoints (e.g., body weight, organ histopathology) to confirm low acute toxicity (LD50 = 104 mg/kg, intraperitoneal) .
Advanced Research Questions
Q. How can contradictory IC50 values for 10-HCPT across cell lines be systematically analyzed?
- Methodological Answer : Perform meta-analysis of IC50 data (e.g., BT-20 vs. MDA-231) by normalizing for variables like cell doubling time, drug uptake efficiency, and baseline topoisomerase I expression (western blot). Use multivariate regression to identify confounding factors .
Q. What strategies resolve discrepancies between in vitro and in vivo efficacy of 10-HCPT?
- Methodological Answer : Compare drug stability in culture media vs. plasma. For example, 10-HCPT’s lactone ring is pH-sensitive; use LC-MS to quantify active vs. inactive forms in both settings. Optimize delivery systems (e.g., liposomal encapsulation) to enhance bioavailability in vivo .
Q. How can researchers differentiate 10-HCPT’s direct topoisomerase I inhibition from off-target effects in transcriptomic studies?
- Methodological Answer : Perform RNA sequencing on treated cells (e.g., HMECs) with and without topoisomerase I knockdown. Use pathway enrichment analysis (e.g., KEGG) to isolate gene expression changes specific to DNA damage response (e.g., p53, ATM/ATR pathways) .
Q. What computational approaches predict 10-HCPT’s binding dynamics with topoisomerase I?
- Methodological Answer : Use molecular docking (e.g., AutoDock Vina) to model 10-HCPT’s interaction with the topoisomerase I-DNA complex. Validate predictions with mutagenesis studies (e.g., mutations in catalytic tyrosine residues) and surface plasmon resonance (SPR) to measure binding affinity .
Q. How do researchers address variability in 10-HCPT’s anti-tumor activity across preclinical models (e.g., xenografts vs. PDX models)?
- Methodological Answer : Use patient-derived xenografts (PDX) with documented topoisomerase I expression levels. Stratify results by tumor subtype and compare response rates with xenograft data. Apply statistical tools (e.g., ANOVA) to assess model-specific biases .
Q. What methodologies quantify 10-HCPT’s impact on viral replication in dual-mechanism studies (e.g., antiviral + anticancer)?
- Methodological Answer : Treat HSV-1-infected cells with 10-HCPT and quantify viral DNA via qPCR. Normalize results to cytotoxicity (MTT assay) and compare with untreated controls. For example, 10-HCPT reduces HSV-1 DNA copies by >50% at 8 hours post-infection (p < 0.0001) .
Properties
IUPAC Name |
(19S)-19-ethyl-7,19-dihydroxy-17-oxa-3,13-diazapentacyclo[11.8.0.02,11.04,9.015,20]henicosa-1(21),2(11),3,5,7,9,15(20)-heptaene-14,18-dione | |
---|---|---|
Source | PubChem | |
URL | https://pubchem.ncbi.nlm.nih.gov | |
Description | Data deposited in or computed by PubChem | |
InChI |
InChI=1S/C20H16N2O5/c1-2-20(26)14-7-16-17-11(5-10-6-12(23)3-4-15(10)21-17)8-22(16)18(24)13(14)9-27-19(20)25/h3-7,23,26H,2,8-9H2,1H3/t20-/m0/s1 | |
Source | PubChem | |
URL | https://pubchem.ncbi.nlm.nih.gov | |
Description | Data deposited in or computed by PubChem | |
InChI Key |
HAWSQZCWOQZXHI-FQEVSTJZSA-N | |
Source | PubChem | |
URL | https://pubchem.ncbi.nlm.nih.gov | |
Description | Data deposited in or computed by PubChem | |
Canonical SMILES |
CCC1(C2=C(COC1=O)C(=O)N3CC4=C(C3=C2)N=C5C=CC(=CC5=C4)O)O | |
Source | PubChem | |
URL | https://pubchem.ncbi.nlm.nih.gov | |
Description | Data deposited in or computed by PubChem | |
Isomeric SMILES |
CC[C@@]1(C2=C(COC1=O)C(=O)N3CC4=C(C3=C2)N=C5C=CC(=CC5=C4)O)O | |
Source | PubChem | |
URL | https://pubchem.ncbi.nlm.nih.gov | |
Description | Data deposited in or computed by PubChem | |
Molecular Formula |
C20H16N2O5 | |
Source | PubChem | |
URL | https://pubchem.ncbi.nlm.nih.gov | |
Description | Data deposited in or computed by PubChem | |
DSSTOX Substance ID |
DTXSID00941444 | |
Record name | 4-Ethyl-4,9-dihydroxy-1H-pyrano[3',4':6,7]indolizino[1,2-b]quinoline-3,14(4H,12H)-dione | |
Source | EPA DSSTox | |
URL | https://comptox.epa.gov/dashboard/DTXSID00941444 | |
Description | DSSTox provides a high quality public chemistry resource for supporting improved predictive toxicology. | |
Molecular Weight |
364.4 g/mol | |
Source | PubChem | |
URL | https://pubchem.ncbi.nlm.nih.gov | |
Description | Data deposited in or computed by PubChem | |
CAS No. |
19685-09-7 | |
Record name | Hydroxycamptothecin | |
Source | CAS Common Chemistry | |
URL | https://commonchemistry.cas.org/detail?cas_rn=19685-09-7 | |
Description | CAS Common Chemistry is an open community resource for accessing chemical information. Nearly 500,000 chemical substances from CAS REGISTRY cover areas of community interest, including common and frequently regulated chemicals, and those relevant to high school and undergraduate chemistry classes. This chemical information, curated by our expert scientists, is provided in alignment with our mission as a division of the American Chemical Society. | |
Explanation | The data from CAS Common Chemistry is provided under a CC-BY-NC 4.0 license, unless otherwise stated. | |
Record name | 10-Hydroxycamptothecin | |
Source | ChemIDplus | |
URL | https://pubchem.ncbi.nlm.nih.gov/substance/?source=chemidplus&sourceid=0019685097 | |
Description | ChemIDplus is a free, web search system that provides access to the structure and nomenclature authority files used for the identification of chemical substances cited in National Library of Medicine (NLM) databases, including the TOXNET system. | |
Record name | 10-hydroxycamptothecin | |
Source | DrugBank | |
URL | https://www.drugbank.ca/drugs/DB12385 | |
Description | The DrugBank database is a unique bioinformatics and cheminformatics resource that combines detailed drug (i.e. chemical, pharmacological and pharmaceutical) data with comprehensive drug target (i.e. sequence, structure, and pathway) information. | |
Explanation | Creative Common's Attribution-NonCommercial 4.0 International License (http://creativecommons.org/licenses/by-nc/4.0/legalcode) | |
Record name | 10-Hydroxycamptothecin | |
Source | DTP/NCI | |
URL | https://dtp.cancer.gov/dtpstandard/servlet/dwindex?searchtype=NSC&outputformat=html&searchlist=107124 | |
Description | The NCI Development Therapeutics Program (DTP) provides services and resources to the academic and private-sector research communities worldwide to facilitate the discovery and development of new cancer therapeutic agents. | |
Explanation | Unless otherwise indicated, all text within NCI products is free of copyright and may be reused without our permission. Credit the National Cancer Institute as the source. | |
Record name | 4-Ethyl-4,9-dihydroxy-1H-pyrano[3',4':6,7]indolizino[1,2-b]quinoline-3,14(4H,12H)-dione | |
Source | EPA DSSTox | |
URL | https://comptox.epa.gov/dashboard/DTXSID00941444 | |
Description | DSSTox provides a high quality public chemistry resource for supporting improved predictive toxicology. | |
Record name | 10-Hydroxycamptothecin | |
Source | European Chemicals Agency (ECHA) | |
URL | https://echa.europa.eu/information-on-chemicals | |
Description | The European Chemicals Agency (ECHA) is an agency of the European Union which is the driving force among regulatory authorities in implementing the EU's groundbreaking chemicals legislation for the benefit of human health and the environment as well as for innovation and competitiveness. | |
Explanation | Use of the information, documents and data from the ECHA website is subject to the terms and conditions of this Legal Notice, and subject to other binding limitations provided for under applicable law, the information, documents and data made available on the ECHA website may be reproduced, distributed and/or used, totally or in part, for non-commercial purposes provided that ECHA is acknowledged as the source: "Source: European Chemicals Agency, http://echa.europa.eu/". Such acknowledgement must be included in each copy of the material. ECHA permits and encourages organisations and individuals to create links to the ECHA website under the following cumulative conditions: Links can only be made to webpages that provide a link to the Legal Notice page. | |
Record name | 10-HYDROXYCAMPTOTHECIN | |
Source | FDA Global Substance Registration System (GSRS) | |
URL | https://gsrs.ncats.nih.gov/ginas/app/beta/substances/9Z01632KRV | |
Description | The FDA Global Substance Registration System (GSRS) enables the efficient and accurate exchange of information on what substances are in regulated products. Instead of relying on names, which vary across regulatory domains, countries, and regions, the GSRS knowledge base makes it possible for substances to be defined by standardized, scientific descriptions. | |
Explanation | Unless otherwise noted, the contents of the FDA website (www.fda.gov), both text and graphics, are not copyrighted. They are in the public domain and may be republished, reprinted and otherwise used freely by anyone without the need to obtain permission from FDA. Credit to the U.S. Food and Drug Administration as the source is appreciated but not required. | |
Retrosynthesis Analysis
AI-Powered Synthesis Planning: Our tool employs the Template_relevance Pistachio, Template_relevance Bkms_metabolic, Template_relevance Pistachio_ringbreaker, Template_relevance Reaxys, Template_relevance Reaxys_biocatalysis model, leveraging a vast database of chemical reactions to predict feasible synthetic routes.
One-Step Synthesis Focus: Specifically designed for one-step synthesis, it provides concise and direct routes for your target compounds, streamlining the synthesis process.
Accurate Predictions: Utilizing the extensive PISTACHIO, BKMS_METABOLIC, PISTACHIO_RINGBREAKER, REAXYS, REAXYS_BIOCATALYSIS database, our tool offers high-accuracy predictions, reflecting the latest in chemical research and data.
Strategy Settings
Precursor scoring | Relevance Heuristic |
---|---|
Min. plausibility | 0.01 |
Model | Template_relevance |
Template Set | Pistachio/Bkms_metabolic/Pistachio_ringbreaker/Reaxys/Reaxys_biocatalysis |
Top-N result to add to graph | 6 |
Feasible Synthetic Routes
Disclaimer and Information on In-Vitro Research Products
Please be aware that all articles and product information presented on BenchChem are intended solely for informational purposes. The products available for purchase on BenchChem are specifically designed for in-vitro studies, which are conducted outside of living organisms. In-vitro studies, derived from the Latin term "in glass," involve experiments performed in controlled laboratory settings using cells or tissues. It is important to note that these products are not categorized as medicines or drugs, and they have not received approval from the FDA for the prevention, treatment, or cure of any medical condition, ailment, or disease. We must emphasize that any form of bodily introduction of these products into humans or animals is strictly prohibited by law. It is essential to adhere to these guidelines to ensure compliance with legal and ethical standards in research and experimentation.