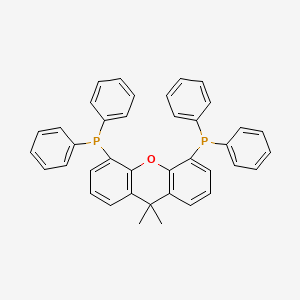
Xantphos
Overview
Description
Xantphos (4,5-bis(diphenylphosphino)-9,9-dimethylxanthene) is a bidentate phosphine ligand widely employed in transition metal catalysis. Its rigid xanthene backbone and flexible coordination environment enable a wide bite angle (∼108°), which enhances catalytic activity in reactions requiring large coordination spheres, such as carbonylation, cross-coupling, and cycloadditions . This compound forms stable complexes with metals like Pd, Ni, Rh, and Cu, facilitating diverse transformations, including aryl bromide carbonylation , Negishi coupling , and pyridine synthesis via Ni-catalyzed cycloaddition . Its commercial availability and adaptability to steric and electronic modifications (e.g., HN-xantphos, Nithis compound) further broaden its utility .
Preparation Methods
Xantphos is typically synthesized through a double directed lithiation of 9,9-dimethylxanthene using sec-butyllithium, followed by treatment with chlorodiphenylphosphine . The reaction conditions involve low temperatures to ensure the stability of the intermediates and the final product. Industrial production methods often involve similar synthetic routes but are optimized for large-scale production, ensuring high yield and purity.
Chemical Reactions Analysis
Xantphos undergoes a variety of chemical reactions, primarily due to its role as a ligand in metal-catalyzed processes. Some of the key reactions include:
Cross-Coupling Reactions: This compound-ligated palladium complexes are highly effective in C–N, C–S, and C–C cross-coupling reactions.
Hydroformylation: This compound is used in rhodium-catalyzed hydroformylation of alkenes, leading to the formation of aldehydes.
Cycloaddition Reactions: This compound nickel π-complexes are competent in cycloaddition reactions.
Common reagents used in these reactions include palladium, rhodium, and nickel complexes, often in the presence of various solvents and under specific temperature and pressure conditions. The major products formed from these reactions are typically aldehydes, coupled products, and cycloadducts.
Scientific Research Applications
Xantphos has a wide range of applications in scientific research, particularly in the fields of chemistry and catalysis. Some notable applications include:
Homogeneous Catalysis: This compound is extensively used as a ligand in homogeneous catalysis, facilitating reactions such as hydroformylation, alkoxycarbonylation, and cross-coupling reactions.
CO2 Hydrogenation: This compound-based macroligands have been employed in the catalytic hydrogenation of carbon dioxide to formic acid, showcasing its potential in sustainable chemistry.
Material Science: This compound derivatives are used in the development of porous organic polymers and other advanced materials.
Mechanism of Action
The mechanism by which Xantphos exerts its effects is primarily through its role as a ligand in metal-catalyzed reactions. The wide bite angle of this compound allows for the formation of stable metal-ligand complexes, which are crucial for catalytic activity. In hydroformylation reactions, for example, this compound facilitates the coordination of the metal center to the substrate, promoting the formation of the desired product .
Comparison with Similar Compounds
Comparison with Similar Bidentate Ligands in Catalytic Reactions
Hydroformylation: Xantphos vs. Biphephos and A4N3
In Rh-catalyzed hydroformylation, this compound demonstrates distinct selectivity compared to Biphephos and A4N3. A study comparing Rh–this compound, Rh–Biphephos, and Rh–A4N3 in oleonitrile isomerization-hydroformylation revealed:
Entry | Ligand | Conversion (%) | Linear:Branched Aldehyde Ratio | |
---|---|---|---|---|
1 | Biphephos | 95 | 85:15 | |
2 | A4N3 | 89 | 78:22 | |
3 | This compound | 76 | 70:30 |
Biphephos outperformed this compound in both conversion and linear selectivity due to its smaller bite angle (∼107° vs. This compound’ 108°), which optimizes substrate alignment. However, this compound excels in reactions requiring steric flexibility, such as carbonylation .
Carbonylation: this compound vs. DPEphos and dppf
In Pd-catalyzed aryl bromide carbonylation, this compound outperforms DPEphos and dppf. Under identical conditions, this compound achieved 98% conversion to methyl esters within 2 hours, while DPEphos and dppf (similar bite angles) showed negligible activity . The flexibility of this compound’ xanthene backbone allows cis-coordination in Pd-acyl intermediates, a critical factor for catalytic turnover .
Cross-Coupling: this compound vs. Monodentate Ligands
This compound accelerates reductive elimination in Pd-catalyzed amidative cross-coupling compared to monodentate ligands. Its bite angle promotes faster C–N bond formation, achieving 90% yield versus <50% for monodentate analogues . However, excessive this compound can form inactive Pd(this compound)₂ species, reducing catalytic efficiency .
Photoluminescence Quantum Yield (PLQY)
Complex | PLQY (%) | λₑₘ (nm) | |
---|---|---|---|
[Cu(this compound)(6,6′-Me₂bpy)][PF₆] | 37 | 556 | |
[Cu(POP)(6,6′-Me₂bpy)][PF₆] | 28 | 589 | |
[Cu(dpephos)(Htbz)][PF₆] | 15 | 605 |
This compound complexes exhibit higher PLQY and blue-shifted emission due to enhanced π-stacking and rigid coordination, which minimize non-radiative decay .
Electrochemical Stability
Oxidation potentials (E₁/₂ₒₓ) for this compound complexes increase with steric bulk:
- [Cu(this compound)(bpy)][PF₆]: +0.76 V
- [Cu(this compound)(6-Mebpy)][PF₆]: +0.85 V
- [Cu(this compound)(6,6′-Me₂bpy)][PF₆]: +0.96 V
This trend contrasts with dpephos complexes, where Cu participation in frontier orbitals is more pronounced (37% vs. 6% for this compound), affecting charge-transfer dynamics .
Specialized this compound Derivatives
HN-Xantphos vs. This compound
HN-xantphos (N–H functionalized) shows weaker emission in solution due to ligand redistribution but matches this compound’ solid-state PLQY (e.g., 37% for [Cu(HN-xantphos)(6,6′-Me₂bpy)][PF₆]). However, its instability in polar solvents limits applications .
Nithis compound vs. This compound
Nithis compound, featuring a deprotonatable NH group, enables room-temperature Pd-catalyzed cross-couplings (91% yield with aryl chlorides), whereas this compound fails (0% yield). The NH group facilitates oxidative addition via heterobimetallic Pd–Ni interactions .
Critical Analysis and Key Trends
- Bite Angle and Flexibility : this compound’ wide, adjustable bite angle (108–120°) is pivotal in carbonylation and cycloadditions, outperforming rigid ligands like dppf .
- Steric Effects : Substituents on ancillary ligands (e.g., 6,6′-Me₂bpy) enhance PLQY in Cu complexes by reducing excited-state distortion .
- Ligand Synergy : In Pd-catalyzed desaturation, this compound requires Cy-JohnPhos for optimal activity, highlighting the need for complementary ligands in complex mechanisms .
Biological Activity
Xantphos, a bidentate phosphine ligand, is recognized for its significant role in catalysis and biological activity. This article explores the biological implications of this compound, focusing on its mechanisms, applications, and case studies that highlight its effectiveness in various chemical reactions.
Overview of this compound
This compound, also known as 4,5-bis(diphenylphosphino)-9,9-dimethylxanthene, is a versatile ligand used primarily in palladium-catalyzed reactions. Its unique structure allows it to stabilize metal centers, facilitating various catalytic processes. The ligand's biological activity is increasingly being studied for its potential therapeutic applications.
Mechanisms of Biological Activity
The biological activity of this compound can be attributed to its ability to form stable complexes with metal catalysts, which in turn influence biological pathways. Some key mechanisms include:
- Catalytic Activity : this compound enhances the efficiency of palladium complexes in carbonylation reactions, which are crucial for synthesizing biologically active compounds .
- Metal Coordination : The ligand's ability to coordinate with metals like palladium and rhodium allows it to participate in various organic transformations that can lead to biologically relevant products .
- Electrophilic Activation : this compound can activate electrophiles through its coordination with metal centers, making it essential in processes like allylic alkylation .
Case Study 1: Allylic Alkylation
A study investigated the role of this compound in the allylic alkylation of α-amino esters. The research demonstrated that the presence of this compound significantly increased the yield of α-quaternary α-amino esters by stabilizing the dirhodium complex involved in the reaction. Without this compound, the reaction stalled at an intermediate stage .
Case Study 2: Carbonylation Reactions
Research highlighted that the [PdCl2(this compound)] complex exhibited high catalytic activity for the methoxycarbonylation of iodobenzene. The optimized conditions led to turnover frequencies (TOFs) exceeding 300,000 h, showcasing this compound's effectiveness in facilitating carbonylation reactions at low concentrations .
Data Table: Comparison of Catalytic Activities
Reaction Type | Catalyst Used | TOF (h) | Yield (%) |
---|---|---|---|
Allylic Alkylation | Rh/Xantphos | - | High |
Methoxycarbonylation | PdCl2(this compound) | >300,000 | High |
Coupling Reactions | Pd/Xantphos | - | Moderate |
Q & A
Basic Research Questions
Q. What structural features make Xantphos effective in transition metal catalysis?
this compound features a rigid xanthene backbone with two diphenylphosphine groups, creating a wide natural bite angle (~108°). This geometry stabilizes metal centers (e.g., Pd, Ru, Ni) by reducing steric strain during catalytic cycles, while the electron-rich phosphine groups enhance oxidative addition and transmetalation steps. The backbone’s rigidity also prevents ligand dissociation, maintaining catalytic integrity under harsh conditions .
Q. What are the historical milestones and key catalytic applications of this compound?
this compound was first synthesized in 1986 by Denis P. Curran and gained prominence in Pd-catalyzed cross-coupling reactions (e.g., Suzuki-Miyaura, Heck, and Buchwald-Hartwig aminations). Its broad applicability extends to Ru-catalyzed alcohol amination and Ni-mediated alkyne cycloadditions. These reactions benefit from this compound’s ability to stabilize low-coordinate metal intermediates, enabling high turnover numbers (TONs) and chemoselectivity .
Q. How does this compound compare to other bidentate phosphine ligands in cross-coupling reactions?
this compound outperforms monodentate ligands (e.g., PPh₃) and narrower bite-angle ligands (e.g., DPPF) in reactions requiring steric and electronic stabilization. For example, in Pd-catalyzed C–N coupling, this compound’s wide bite angle accelerates reductive elimination by 10–100× compared to BINAP, as shown in kinetic studies using ³¹P NMR and reaction calorimetry .
Advanced Research Questions
Q. How does this compound exhibit fluxional behavior in Ru-catalyzed systems?
In RuHCl(CO)(PPh₃)(this compound) complexes, VT-NMR studies reveal temperature-dependent fluxionality of the xanthene backbone. The energy barrier for conformational "flipping" is ~55.8 kJ/mol (ΔS ≈ -46.1 J/mol·K), indicating minimal entropy change and no ligand dissociation. This dynamic behavior enables adaptive coordination during catalytic alcohol amination, as demonstrated by crystallographic and kinetic data .
Q. Why does excess this compound suppress activity in Pd-catalyzed C–N bond formation?
At high ligand-to-Pd ratios (>1.5:1), this compound forms the bis-ligated Pd(this compound)₂ species, which exhibits low catalytic activity due to slow dissociation kinetics (koff ~10⁻⁴ s⁻¹). This inertness was confirmed via magnetometry transfer experiments and comparative studies with soluble analogs (e.g., 4,7-di-tert-butyl this compound). The equilibrium between active (this compound)Pd⁰ and inactive Pd(this compound)₂ governs reaction rates .
Q. What role does this compound play in binuclear Pd(I) catalysis for hydroformylation?
In Pd(I)–Pd(I) systems, this compound facilitates a binuclear rate-limiting step involving Pd-acyl and Pd-hydride intermediates. Job plot analyses (molar ratio studies) and kinetic isotope effects (KIE = 2.1) confirm a 2:1 Pd:this compound stoichiometry in the active species. Excess iodide (2–4 eq.) optimizes activity by stabilizing the Pd-hydride intermediate, while deviations reduce turnover frequencies by >50% .
Q. How can this compound derivatives enable mechanistic tracking via mass spectrometry?
Disulfonated this compound (dianionic) serves as a charged tag for real-time ESI-MS analysis. This modification allows detection of transient intermediates (e.g., Cu–this compound adducts) and metal-transfer events (e.g., Cu→Pd) in cross-coupling reactions. The method has quantified ligand exchange rates (kex ~10³ M⁻¹s⁻¹) and identified off-cycle species in Ni-catalyzed systems .
Q. What computational insights explain this compound’s regioselectivity in hydroformylation?
ONIOM hybrid calculations show that this compound’s wide bite angle in Rh complexes promotes diequatorial coordination of CO ligands, favoring linear aldehyde formation (n:iso = 42:1). Transition-state modeling aligns with experimental regioselectivity (n-nonanal:iso-nonanal = 52:1) but underestimates activation barriers by ~5 kcal/mol, highlighting challenges in modeling π-backbonding effects .
Properties
IUPAC Name |
(5-diphenylphosphanyl-9,9-dimethylxanthen-4-yl)-diphenylphosphane | |
---|---|---|
Source | PubChem | |
URL | https://pubchem.ncbi.nlm.nih.gov | |
Description | Data deposited in or computed by PubChem | |
InChI |
InChI=1S/C39H32OP2/c1-39(2)33-25-15-27-35(41(29-17-7-3-8-18-29)30-19-9-4-10-20-30)37(33)40-38-34(39)26-16-28-36(38)42(31-21-11-5-12-22-31)32-23-13-6-14-24-32/h3-28H,1-2H3 | |
Source | PubChem | |
URL | https://pubchem.ncbi.nlm.nih.gov | |
Description | Data deposited in or computed by PubChem | |
InChI Key |
CXNIUSPIQKWYAI-UHFFFAOYSA-N | |
Source | PubChem | |
URL | https://pubchem.ncbi.nlm.nih.gov | |
Description | Data deposited in or computed by PubChem | |
Canonical SMILES |
CC1(C2=C(C(=CC=C2)P(C3=CC=CC=C3)C4=CC=CC=C4)OC5=C1C=CC=C5P(C6=CC=CC=C6)C7=CC=CC=C7)C | |
Source | PubChem | |
URL | https://pubchem.ncbi.nlm.nih.gov | |
Description | Data deposited in or computed by PubChem | |
Molecular Formula |
C39H32OP2 | |
Source | PubChem | |
URL | https://pubchem.ncbi.nlm.nih.gov | |
Description | Data deposited in or computed by PubChem | |
DSSTOX Substance ID |
DTXSID20348350 | |
Record name | Xantphos | |
Source | EPA DSSTox | |
URL | https://comptox.epa.gov/dashboard/DTXSID20348350 | |
Description | DSSTox provides a high quality public chemistry resource for supporting improved predictive toxicology. | |
Molecular Weight |
578.6 g/mol | |
Source | PubChem | |
URL | https://pubchem.ncbi.nlm.nih.gov | |
Description | Data deposited in or computed by PubChem | |
CAS No. |
161265-03-8 | |
Record name | 1,1′-(9,9-Dimethyl-9H-xanthene-4,5-diyl)bis[1,1-diphenylphosphine] | |
Source | CAS Common Chemistry | |
URL | https://commonchemistry.cas.org/detail?cas_rn=161265-03-8 | |
Description | CAS Common Chemistry is an open community resource for accessing chemical information. Nearly 500,000 chemical substances from CAS REGISTRY cover areas of community interest, including common and frequently regulated chemicals, and those relevant to high school and undergraduate chemistry classes. This chemical information, curated by our expert scientists, is provided in alignment with our mission as a division of the American Chemical Society. | |
Explanation | The data from CAS Common Chemistry is provided under a CC-BY-NC 4.0 license, unless otherwise stated. | |
Record name | Xantphos | |
Source | ChemIDplus | |
URL | https://pubchem.ncbi.nlm.nih.gov/substance/?source=chemidplus&sourceid=0161265038 | |
Description | ChemIDplus is a free, web search system that provides access to the structure and nomenclature authority files used for the identification of chemical substances cited in National Library of Medicine (NLM) databases, including the TOXNET system. | |
Record name | Xantphos | |
Source | EPA DSSTox | |
URL | https://comptox.epa.gov/dashboard/DTXSID20348350 | |
Description | DSSTox provides a high quality public chemistry resource for supporting improved predictive toxicology. | |
Record name | Phosphine, 1,1'-(9,9-dimethyl-9H-xanthene-4,5-diyl)bis[1,1-diphenyl | |
Source | European Chemicals Agency (ECHA) | |
URL | https://echa.europa.eu/substance-information/-/substanceinfo/100.118.008 | |
Description | The European Chemicals Agency (ECHA) is an agency of the European Union which is the driving force among regulatory authorities in implementing the EU's groundbreaking chemicals legislation for the benefit of human health and the environment as well as for innovation and competitiveness. | |
Explanation | Use of the information, documents and data from the ECHA website is subject to the terms and conditions of this Legal Notice, and subject to other binding limitations provided for under applicable law, the information, documents and data made available on the ECHA website may be reproduced, distributed and/or used, totally or in part, for non-commercial purposes provided that ECHA is acknowledged as the source: "Source: European Chemicals Agency, http://echa.europa.eu/". Such acknowledgement must be included in each copy of the material. ECHA permits and encourages organisations and individuals to create links to the ECHA website under the following cumulative conditions: Links can only be made to webpages that provide a link to the Legal Notice page. | |
Record name | XANTPHOS | |
Source | FDA Global Substance Registration System (GSRS) | |
URL | https://gsrs.ncats.nih.gov/ginas/app/beta/substances/NMU72MOG9B | |
Description | The FDA Global Substance Registration System (GSRS) enables the efficient and accurate exchange of information on what substances are in regulated products. Instead of relying on names, which vary across regulatory domains, countries, and regions, the GSRS knowledge base makes it possible for substances to be defined by standardized, scientific descriptions. | |
Explanation | Unless otherwise noted, the contents of the FDA website (www.fda.gov), both text and graphics, are not copyrighted. They are in the public domain and may be republished, reprinted and otherwise used freely by anyone without the need to obtain permission from FDA. Credit to the U.S. Food and Drug Administration as the source is appreciated but not required. | |
Retrosynthesis Analysis
AI-Powered Synthesis Planning: Our tool employs the Template_relevance Pistachio, Template_relevance Bkms_metabolic, Template_relevance Pistachio_ringbreaker, Template_relevance Reaxys, Template_relevance Reaxys_biocatalysis model, leveraging a vast database of chemical reactions to predict feasible synthetic routes.
One-Step Synthesis Focus: Specifically designed for one-step synthesis, it provides concise and direct routes for your target compounds, streamlining the synthesis process.
Accurate Predictions: Utilizing the extensive PISTACHIO, BKMS_METABOLIC, PISTACHIO_RINGBREAKER, REAXYS, REAXYS_BIOCATALYSIS database, our tool offers high-accuracy predictions, reflecting the latest in chemical research and data.
Strategy Settings
Precursor scoring | Relevance Heuristic |
---|---|
Min. plausibility | 0.01 |
Model | Template_relevance |
Template Set | Pistachio/Bkms_metabolic/Pistachio_ringbreaker/Reaxys/Reaxys_biocatalysis |
Top-N result to add to graph | 6 |
Feasible Synthetic Routes
Disclaimer and Information on In-Vitro Research Products
Please be aware that all articles and product information presented on BenchChem are intended solely for informational purposes. The products available for purchase on BenchChem are specifically designed for in-vitro studies, which are conducted outside of living organisms. In-vitro studies, derived from the Latin term "in glass," involve experiments performed in controlled laboratory settings using cells or tissues. It is important to note that these products are not categorized as medicines or drugs, and they have not received approval from the FDA for the prevention, treatment, or cure of any medical condition, ailment, or disease. We must emphasize that any form of bodily introduction of these products into humans or animals is strictly prohibited by law. It is essential to adhere to these guidelines to ensure compliance with legal and ethical standards in research and experimentation.