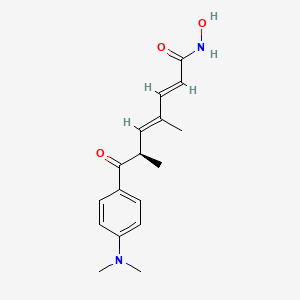
trichostatin A
Overview
Description
Trichostatin A is an organic compound that serves as an antifungal antibiotic. It selectively inhibits the class I and II mammalian histone deacetylase families of enzymes, but not class III histone deacetylases (sirtuins). This compound is known for its ability to alter gene expression by interfering with the removal of acetyl groups from histones, thereby affecting the ability of DNA transcription factors to access DNA molecules inside chromatin .
Mechanism of Action
Mode of Action
TSA interferes with the removal of acetyl groups from histones by inhibiting HDACs . This alters the ability of DNA transcription factors to access the DNA molecules inside chromatin . As a result, TSA can be used to alter gene expression .
Biochemical Pathways
By inhibiting HDACs, TSA affects the acetylation status of histones, thereby influencing the chromatin structure and gene expression . This can lead to various downstream effects, including the promotion of apoptosis-related genes, which can slow the progression of cancer .
Pharmacokinetics
After administration, TSA is rapidly absorbed and detectable in plasma within 2 minutes . The maximum concentration (Cmax) of 40 μg/ml and 8 ng/ml occurs within 5 minutes, followed by a rapid exponential decay in plasma TSA concentration with a half-life (t1/2) of 6.3 minutes and 9.6 minutes for high and low doses, respectively .
Result of Action
TSA has potential as an anti-cancer drug . It promotes the expression of apoptosis-related genes, leading to cancerous cells surviving at lower rates, thus slowing the progression of cancer . Other mechanisms may include the activity of HDIs to induce cell differentiation, thus acting to “mature” some of the de-differentiated cells found in tumors .
Biochemical Analysis
Biochemical Properties
Trichostatin A selectively inhibits the removal of acetyl groups from the amino-terminal lysine residues of core histones . This modulates the access of transcription factors to the underlying genomic DNA . It interacts with HDACs 1, 3, 4, 6, and 10 .
Cellular Effects
This compound inhibits the eukaryotic cell cycle during the beginning of the growth stage . It can alter gene expression by interfering with the removal of acetyl groups from histones . In human Jurkat T cells, this compound arrests cell cycle progression in G1 .
Molecular Mechanism
This compound exerts its effects at the molecular level by inhibiting HDACs . This inhibition disrupts the removal of acetyl groups from histones, altering the ability of DNA transcription factors to access the DNA molecules inside chromatin .
Temporal Effects in Laboratory Settings
This compound has been shown to increase mitochondrial reactive oxygen species production, triggering apoptosis in human breast cancer cells . This effect is dependent on the production of mitochondria-derived reactive oxygen species, which is derived from impaired mitochondrial respiratory chain .
Metabolic Pathways
This compound is involved in the metabolic pathway of histone deacetylation . It inhibits HDACs, which are enzymes that remove acetyl groups from an ε-N-acetyl lysine amino acid on a histone .
Subcellular Localization
This compound targets the mitochondrial respiratory chain, increasing mitochondrial reactive oxygen species production . This suggests that this compound may localize to the mitochondria within cells .
Preparation Methods
Trichostatin A is produced by the bacterium Streptomyces hygroscopicus. The synthetic route involves the preparation of a 2 mg/ml stock solution by adding 500 µl of dimethyl sulfoxide to 1 mg of this compound. The mixture is then vortexed and can be used immediately or stored at -20°C. Further dilutions can be prepared using sterile, endotoxin-free water or aqueous buffers .
Chemical Reactions Analysis
Trichostatin A undergoes various chemical reactions, including:
Oxidation: This compound exhibits antioxidant activity by suppressing oxidative stress through multiple mechanisms.
Reduction: It can be reduced to its corresponding amine derivative under specific conditions.
Substitution: this compound can undergo substitution reactions, particularly at the hydroxamic acid moiety.
Common reagents used in these reactions include oxidizing agents like hydrogen peroxide and reducing agents like sodium borohydride. The major products formed from these reactions depend on the specific conditions and reagents used.
Scientific Research Applications
Trichostatin A has a wide range of scientific research applications:
Chemistry: It is used as a histone deacetylase inhibitor to study epigenetic modifications and gene expression.
Industry: this compound is used in the development of new therapeutic agents targeting histone deacetylases.
Comparison with Similar Compounds
Trichostatin A is compared with other histone deacetylase inhibitors such as vorinostat (suberoylanilide hydroxamic acid). Both compounds inhibit histone deacetylases, but this compound is more selective for class I and II histone deacetylases, while vorinostat also targets class III histone deacetylases . Other similar compounds include curcumin, which also exhibits histone deacetylase inhibitory activity and has been shown to have significant apoptotic effects in cancer cells .
Similar Compounds
- Vorinostat (suberoylanilide hydroxamic acid)
- Curcumin
- Sodium butyrate
- Valproic acid
This compound stands out due to its high selectivity and potency as a histone deacetylase inhibitor, making it a valuable tool in epigenetic research and potential therapeutic applications.
Biological Activity
Trichostatin A (TSA) is a potent histone deacetylase (HDAC) inhibitor with significant biological activity, particularly in cancer research and developmental biology. Originally derived from the fungus Streptomyces hygroscopicus, TSA has emerged as a crucial tool in epigenetic studies and therapeutic applications. This article explores the biological activity of TSA, highlighting its mechanisms of action, effects on various cell types, and implications for clinical use.
TSA functions primarily as a pan-HDAC inhibitor, selectively targeting class I and II HDACs. It operates by chelating zinc ions in the active sites of these enzymes, leading to increased acetylation of histones and non-histone proteins. This alteration in acetylation status impacts gene expression, cellular differentiation, and apoptosis.
- Inhibition Potency : TSA exhibits an inhibitory constant of 3.4 nM and half-maximal inhibitory concentration (IC50) values ranging from 4.99 nM to 27.6 nM across various HDAC isoforms (HDAC1, 3, 4, 6, 10) .
Cancer Cell Lines
TSA has been extensively studied for its effects on various cancer cell lines:
- Induction of Apoptosis : In prostate cancer cells, TSA induces apoptosis through intrinsic pathways. It has been shown to downregulate survivin, a protein that inhibits apoptosis .
- Cell Cycle Arrest : TSA promotes cell cycle arrest in several tumor types, including breast and bladder cancers . For instance, in bladder cancer cells, TSA triggers apoptosis via intrinsic mechanisms at early phases and downregulates Sp1-survivin at later phases .
- Diverse Cancer Types : The compound has demonstrated effectiveness against breast cancer (IC50 = 29-400 nM), melanoma, and osteosarcoma cells . In osteosarcoma specifically, TSA promotes apoptosis through activation of the p53 signaling pathway .
Developmental Biology
TSA's role extends beyond oncology; it also influences developmental processes:
- Embryonic Germ Cell Induction : TSA accelerates the dedifferentiation of primordial germ cells into embryonic germ cells .
- Somatic Cell Nuclear Transfer (SCNT) : Studies indicate that TSA enhances nuclear remodeling in SCNT-generated embryos by improving histone acetylation and DNA replication complex formation . This effect is crucial for improving cloning efficiency.
Case Studies
-
Osteosarcoma Study :
- Objective : To assess the impact of TSA on HDAC activity in osteosarcoma.
- Results : TSA significantly inhibited MG63 cell growth and promoted apoptosis in a dose-dependent manner through p53 pathway activation.
- : Class I HDACs are central to osteosarcoma pathogenesis; thus, HDAC inhibitors like TSA hold therapeutic promise .
- Bladder Cancer Study :
Summary Table of Biological Activities
Properties
CAS No. |
58880-19-6 |
---|---|
Molecular Formula |
C17H22N2O3 |
Molecular Weight |
302.37 g/mol |
IUPAC Name |
(2E,4E)-7-[4-(dimethylamino)phenyl]-N-hydroxy-4,6-dimethyl-7-oxohepta-2,4-dienamide |
InChI |
InChI=1S/C17H22N2O3/c1-12(5-10-16(20)18-22)11-13(2)17(21)14-6-8-15(9-7-14)19(3)4/h5-11,13,22H,1-4H3,(H,18,20)/b10-5+,12-11+ |
InChI Key |
RTKIYFITIVXBLE-WKWSCTOISA-N |
SMILES |
CC(C=C(C)C=CC(=O)NO)C(=O)C1=CC=C(C=C1)N(C)C |
Isomeric SMILES |
CC(/C=C(\C)/C=C/C(=O)NO)C(=O)C1=CC=C(C=C1)N(C)C |
Canonical SMILES |
CC(C=C(C)C=CC(=O)NO)C(=O)C1=CC=C(C=C1)N(C)C |
Appearance |
Solid powder |
Key on ui other cas no. |
58880-19-6 |
Pictograms |
Irritant |
Purity |
>98% (or refer to the Certificate of Analysis) |
shelf_life |
>2 years if stored properly |
solubility |
Soluble in DMSO, not in water |
storage |
Dry, dark and at 0 - 4 C for short term (days to weeks) or -20 C for long term (months to years). |
Synonyms |
A 300 trichostatin A tricostatin A trychostatin A TSA antibioitc |
Origin of Product |
United States |
Retrosynthesis Analysis
AI-Powered Synthesis Planning: Our tool employs the Template_relevance Pistachio, Template_relevance Bkms_metabolic, Template_relevance Pistachio_ringbreaker, Template_relevance Reaxys, Template_relevance Reaxys_biocatalysis model, leveraging a vast database of chemical reactions to predict feasible synthetic routes.
One-Step Synthesis Focus: Specifically designed for one-step synthesis, it provides concise and direct routes for your target compounds, streamlining the synthesis process.
Accurate Predictions: Utilizing the extensive PISTACHIO, BKMS_METABOLIC, PISTACHIO_RINGBREAKER, REAXYS, REAXYS_BIOCATALYSIS database, our tool offers high-accuracy predictions, reflecting the latest in chemical research and data.
Strategy Settings
Precursor scoring | Relevance Heuristic |
---|---|
Min. plausibility | 0.01 |
Model | Template_relevance |
Template Set | Pistachio/Bkms_metabolic/Pistachio_ringbreaker/Reaxys/Reaxys_biocatalysis |
Top-N result to add to graph | 6 |
Feasible Synthetic Routes
Q1: What is the primary molecular target of Trichostatin A (TSA)?
A1: this compound is a potent and specific inhibitor of histone deacetylases (HDACs) [, , , ].
Q2: How does TSA interact with HDACs?
A2: TSA inhibits HDACs by binding to the enzyme through its hydroxamic acid group. This interaction blocks the enzyme's activity [, ]. The dimethylaniline moiety of TSA is also important for its specific recognition by certain HDACs like TsnB9 [].
Q3: What are the downstream effects of HDAC inhibition by TSA?
A3: HDAC inhibition by TSA leads to increased histone acetylation, altering chromatin structure and influencing gene expression [, , ]. This can result in diverse cellular responses, including:
- Induction of cell cycle arrest: TSA can induce cell cycle arrest at the G1/S [] or G2/M phase [, , , ] in various cancer cell lines. This arrest is often associated with upregulation of cyclin-dependent kinase inhibitors like p21WAF1/CIP1 [, , , , ].
- Induction of apoptosis: TSA has been shown to induce apoptosis in various cancer cell lines [, , , , , , , , , , ]. The mechanisms of apoptosis induction can involve both caspase-dependent pathways (activation of caspase-3, -8, -9) [, , ] and modulation of Bcl-2 family proteins [, ].
- Modulation of specific gene expression: Besides p21WAF1/CIP1, TSA can influence the expression of other genes involved in various cellular processes. For example, TSA can downregulate thymidylate synthase (TS), a key enzyme in nucleotide biosynthesis, leading to increased sensitivity to 5-fluorouracil in cancer cells []. It can also influence the expression of genes involved in inflammation (e.g., CD36, TNF-α, VCAM-1) [], potentially impacting processes like atherosclerosis.
Q4: Does TSA affect the expression of all genes equally?
A4: No, TSA does not affect the expression of all genes equally. Microarray studies comparing TSA with another HDAC inhibitor, CG-1521, showed that these compounds have different gene expression profiles []. This suggests that specific HDAC isoforms might be responsible for regulating distinct sets of genes and that selective HDAC inhibition could lead to more targeted therapeutic effects.
Q5: What is the molecular formula and weight of TSA?
A5: While not explicitly provided in these abstracts, the molecular formula of this compound is C17H22N2O3, and its molecular weight is 302.37 g/mol. This information can be found in chemical databases and research articles focusing on its structure.
Q6: How does the structure of TSA contribute to its HDAC inhibitory activity?
A7: The hydroxamic acid group of TSA is essential for its interaction with the zinc ion in the active site of HDAC enzymes [, ]. Modifications to this group typically lead to a loss or reduction in activity.
Q7: Have any studies investigated the impact of structural modifications on TSA's activity?
A8: Yes, studies investigating valproic acid (VPA) derivatives, structurally similar to TSA, have explored the relationship between structure, HDAC inhibition, and teratogenicity []. These studies highlight the importance of the carboxylic acid function for HDAC inhibition in VPA analogs. Notably, while VPA amides and hydroxamates can cause neural tube defects, they do not inhibit HDACs []. This suggests that the carboxylic acid group, present in VPA but not its amides or hydroxamates, is crucial for HDAC inhibitory activity in this class of compounds. Further research into modified TSA analogs could provide insights into designing more specific and potent HDAC inhibitors.
Q8: What is known about the stability of TSA?
A9: this compound is known to be rapidly metabolized in vivo, limiting its therapeutic application when administered intravenously [].
Q9: Are there any strategies to improve the stability and bioavailability of TSA?
A10: Yes, encapsulating TSA in liposomes, specifically Stealth® liposomes (TSA-lipo), has been shown to improve its stability, enhance tumor accumulation, and increase its anticancer activity in vivo []. This suggests that liposomal formulations could be a promising approach to overcome the limitations associated with TSA's rapid metabolism and improve its therapeutic potential.
Q10: How is TSA metabolized?
A10: While the specific metabolic pathways of TSA are not extensively discussed in these abstracts, its rapid metabolism likely involves enzymatic degradation, potentially via pathways similar to other hydroxamic acid-containing compounds.
Q11: What in vitro models have been used to study TSA?
A11: Researchers have employed various in vitro models to investigate the effects of TSA, including:
- Cancer cell lines: Numerous studies utilize human cancer cell lines, including breast cancer (MCF-7, T47-D, MDA-MB-231, SKBr-3) [, , ], prostate cancer (PC3, LNCaP, 267B1/K-ras) [, , ], lung cancer (A549, NCI-H1299) [, , ], gastric cancer (SGC-7901, MGC-803) [, ], ovarian cancer (skov-3) [], anaplastic thyroid carcinoma (DRO) [], bladder cancer [], and tongue squamous cell carcinoma (SCC-6) [], to assess its anti-proliferative, apoptotic, and gene regulatory effects.
- Primary cell cultures: Studies have also used primary cultures of cells, such as rabbit perichondrial grafts for cartilage regeneration studies [], and human intestinal epithelial cells to investigate the modulation of complement component biosynthesis [].
- Immune cells: TSA's effects on immune responses have been explored in models using natural killer (NK) cells [].
Q12: What in vivo models have been used to study TSA?
A12: In vivo studies investigating the therapeutic potential of TSA have primarily focused on:
- Xenograft models: Researchers have utilized xenograft models, where human cancer cells are implanted into immunodeficient mice, to evaluate TSA's antitumor efficacy. These models have been used for breast cancer (MCF-7, MDA-MB-231) [] and prostate cancer [] studies.
- Animal models of disease: TSA's effects on atherosclerosis were investigated in LDL receptor-deficient (Ldlr−/−) mice, a model prone to developing atherosclerosis when fed a high-fat diet []. Additionally, TSA's impact on conjunctival fibrosis after trabeculectomy was assessed in a rat model [].
- Developmental models: TSA's effects on embryonic development and teratogenicity have been investigated in the NMRI-exencephaly mouse model []. Additionally, its impact on cartilage regeneration was evaluated in a rabbit perichondrial graft model [].
Q13: Are there known mechanisms of resistance to TSA?
A13: While the provided abstracts do not specifically focus on resistance mechanisms to TSA, potential mechanisms could involve:
Q14: What is known about the toxicity and safety profile of TSA?
A17: While the provided research highlights the therapeutic potential of TSA, it's important to note that HDAC inhibitors, including TSA, can exhibit off-target effects and toxicity. For example, in a study investigating the effects of VPA derivatives (structurally similar to TSA) on neural tube defects, researchers found that while VPA amides and hydroxamates can cause these defects, they do not inhibit HDACs []. This suggests that these compounds might exert teratogenic effects through mechanisms independent of HDAC inhibition. Additionally, in a study assessing the impact of TSA on atherosclerosis in LDL receptor-deficient mice, researchers observed exacerbated atherosclerosis in TSA-treated mice, indicating potential pro-atherogenic effects [].
Q15: What strategies are being explored to improve the delivery of TSA to specific targets?
A18: Liposomal encapsulation, exemplified by TSA-lipo, represents a promising drug delivery strategy to enhance TSA's stability, bioavailability, and tumor targeting []. This approach could potentially minimize off-target effects and improve therapeutic efficacy. Further research into targeted delivery systems, such as antibody-drug conjugates or nanoparticle-based approaches, could further enhance TSA's specificity and therapeutic index.
Q16: What biomarkers are being investigated to predict the efficacy of TSA or monitor treatment response?
A16: Several potential biomarkers have emerged from the research on TSA:
Disclaimer and Information on In-Vitro Research Products
Please be aware that all articles and product information presented on BenchChem are intended solely for informational purposes. The products available for purchase on BenchChem are specifically designed for in-vitro studies, which are conducted outside of living organisms. In-vitro studies, derived from the Latin term "in glass," involve experiments performed in controlled laboratory settings using cells or tissues. It is important to note that these products are not categorized as medicines or drugs, and they have not received approval from the FDA for the prevention, treatment, or cure of any medical condition, ailment, or disease. We must emphasize that any form of bodily introduction of these products into humans or animals is strictly prohibited by law. It is essential to adhere to these guidelines to ensure compliance with legal and ethical standards in research and experimentation.