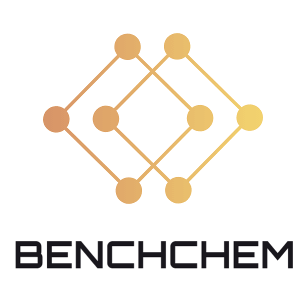
Apicidin
- Click on QUICK INQUIRY to receive a quote from our team of experts.
- With the quality product at a COMPETITIVE price, you can focus more on your research.
Overview
Description
Apicidin is a fungal metabolite and a broad-spectrum antiprotozoal agent . It is a potent inhibitor of histone deacetylases (HDACs), particularly histone deacetylase 1 and 3 (HDAC1 and HDAC3) . It exhibits anti-protozoal activity against apicomplexan metabolite produced by parasites and also possesses anti-proliferative activity against several cancer cell lines .
Synthesis Analysis
Apicidin is produced by certain isolates of Fusarium semitectum . The apicidin biosynthetic pathway involves a non-ribosomal peptide synthetase (NRPS) gene cluster . The NRPS gene, named as apicidin synthetase gene 1 (APS1), along with other genes in the cluster, are required for apicidin production . The cluster gene APS2, a putative transcription factor, regulates the expression of the genes in the cluster .Molecular Structure Analysis
Apicidin is a cyclic tetrapeptide . It has structural similarities to the known histone deacetylase inhibitor apicidin . The molecular formula of Apicidin is C34H49N5O6 .Chemical Reactions Analysis
The apicidin biosynthetic pathway involves several chemical reactions . The apicidin analogues apicidin E, apicidin D2, and apicidin B were identified from chemical analysis of the mutants .Physical And Chemical Properties Analysis
The molecular weight of Apicidin is 623.8 g/mol . The molecular formula of Apicidin is C34H49N5O6 .Scientific Research Applications
Treatment of Acute Myeloid Leukemia (AML)
Apicidin has shown promising therapeutic effects on Acute Myeloid Leukemia (AML) cells . It works by inhibiting cell proliferation, facilitating apoptosis, and inducing myeloid differentiation of AML cells . A potential downstream target of Apicidin, QPCT, has been identified, which exhibits significantly decreased expression in AML samples compared with the normal controls .
Histone Deacetylase Inhibition
Apicidin is a fungus-derived histone deacetylase inhibitor . Histone deacetylases (HDACs) are known to moderate histone acetylation and chromatin folding during gene expression . By inhibiting HDACs, Apicidin can affect gene expression and potentially influence various biological processes .
Antiprotozoal Agent
Apicidin is a broad-spectrum antiprotozoal agent . It has been shown to irreversibly prevent the development of intracellular Apicomplexan parasites in vitro through HDAC inhibition .
Tumor Cell Proliferation Inhibition
Research indicates that Apicidin inhibits tumor cell proliferation through gene expression changes of p21WAF1/Cip1 and gelsolin . It can cause cell cycle arrest in the G1 phase .
Decreasing HIF-1α Protein Levels
Apicidin has been shown to decrease HIF-1α protein levels in human and mouse cell lines . This could potentially have implications in the treatment of diseases where HIF-1α plays a role, such as cancer .
Production by Fungi
Apicidin is a cyclic tetrapeptide produced by certain isolates of Fusarium semitectum . Understanding the production of Apicidin by fungi could potentially lead to improved methods of production for therapeutic use .
Safety And Hazards
properties
{ "Design of the Synthesis Pathway": "The synthesis pathway of Apicidin involves the coupling of a hydroxamic acid and a cyclic peptide. The hydroxamic acid is synthesized separately and then coupled with the cyclic peptide to form Apicidin.", "Starting Materials": [ "L-lysine", "L-leucine", "L-proline", "L-valine", "L-phenylalanine", "L-tyrosine", "L-alanine", "L-serine", "tert-butyl carbamate", "ethyl chloroformate", "triethylamine", "N-hydroxysuccinimide", "N,N'-dicyclohexylcarbodiimide", "2-mercaptoethanol", "acetic anhydride", "sodium hydroxide", "acetic acid", "hydrochloric acid", "methanol", "diethyl ether", "dichloromethane", "dimethylformamide", "dimethyl sulfoxide" ], "Reaction": [ "L-lysine is protected as its tert-butyl carbamate derivative using tert-butyl chloroformate and triethylamine.", "L-leucine, L-proline, L-valine, L-phenylalanine, L-tyrosine, L-alanine, and L-serine are coupled together to form the cyclic peptide using N,N'-dicyclohexylcarbodiimide and N-hydroxysuccinimide as coupling agents.", "The cyclic peptide is then deprotected using 2-mercaptoethanol and acetic acid to remove the tert-butyl carbamate protecting group.", "The hydroxamic acid is synthesized separately by reacting L-lysine with acetic anhydride and sodium hydroxide to form the N-acetyl derivative, which is then reacted with hydroxylamine to form the hydroxamic acid.", "The hydroxamic acid is then coupled with the cyclic peptide using N,N'-dicyclohexylcarbodiimide and N-hydroxysuccinimide as coupling agents.", "The final product, Apicidin, is purified by chromatography using dichloromethane and methanol as solvents." ] } | |
CAS RN |
183506-66-3 |
Product Name |
Apicidin |
Molecular Formula |
C34H49N5O6 |
Molecular Weight |
625.8 g/mol |
IUPAC Name |
methyl 6-[(3S,6S,9S,12R)-3-butan-2-yl-6-[(1-methoxyindol-3-yl)methyl]-2,5,8,11-tetraoxo-1,4,7,10-tetrazabicyclo[10.4.0]hexadecan-9-yl]hexanoate |
InChI |
InChI=1S/C33H47N5O7/c1-5-21(2)29-33(43)37-18-12-11-16-27(37)32(42)34-24(14-7-6-8-17-28(39)44-3)30(40)35-25(31(41)36-29)19-22-20-38(45-4)26-15-10-9-13-23(22)26/h9-10,13,15,20-21,24-25,27,29H,5-8,11-12,14,16-19H2,1-4H3,(H,34,42)(H,35,40)(H,36,41)/t21?,24-,25-,27+,29-/m0/s1 |
InChI Key |
ROUDRKBLRFRFCY-VWIQTCEGSA-N |
Isomeric SMILES |
CC[C@H](C)[C@H]1C(=O)N2CCCC[C@@H]2C(=O)N[C@H](C(=O)N[C@H](C(=O)N1)CC3=CN(C4=CC=CC=C43)OC)CCCCCC(=O)CC |
SMILES |
CCC(C)C1C(=O)N2CCCCC2C(=O)NC(C(=O)NC(C(=O)N1)CC3=CN(C4=CC=CC=C43)OC)CCCCCC(=O)OC |
Canonical SMILES |
CCC(C)C1C(=O)N2CCCCC2C(=O)NC(C(=O)NC(C(=O)N1)CC3=CN(C4=CC=CC=C43)OC)CCCCCC(=O)OC |
Appearance |
Solid powder |
Pictograms |
Acute Toxic |
Purity |
>98% (or refer to the Certificate of Analysis) |
shelf_life |
>5 years if stored properly |
solubility |
Soluble in DMSO, not in water |
storage |
Dry, dark and at 0 - 4 C for short term (days to weeks) or -20 C for long term (months to years). |
synonyms |
apicidin apicidin C cyclo(N-O-methyl-tryptophyl-isoleucyl-pipecolinyl-2-amino-8-oxodecanoyl) |
Origin of Product |
United States |
Retrosynthesis Analysis
AI-Powered Synthesis Planning: Our tool employs the Template_relevance Pistachio, Template_relevance Bkms_metabolic, Template_relevance Pistachio_ringbreaker, Template_relevance Reaxys, Template_relevance Reaxys_biocatalysis model, leveraging a vast database of chemical reactions to predict feasible synthetic routes.
One-Step Synthesis Focus: Specifically designed for one-step synthesis, it provides concise and direct routes for your target compounds, streamlining the synthesis process.
Accurate Predictions: Utilizing the extensive PISTACHIO, BKMS_METABOLIC, PISTACHIO_RINGBREAKER, REAXYS, REAXYS_BIOCATALYSIS database, our tool offers high-accuracy predictions, reflecting the latest in chemical research and data.
Strategy Settings
Precursor scoring | Relevance Heuristic |
---|---|
Min. plausibility | 0.01 |
Model | Template_relevance |
Template Set | Pistachio/Bkms_metabolic/Pistachio_ringbreaker/Reaxys/Reaxys_biocatalysis |
Top-N result to add to graph | 6 |
Feasible Synthetic Routes
Disclaimer and Information on In-Vitro Research Products
Please be aware that all articles and product information presented on BenchChem are intended solely for informational purposes. The products available for purchase on BenchChem are specifically designed for in-vitro studies, which are conducted outside of living organisms. In-vitro studies, derived from the Latin term "in glass," involve experiments performed in controlled laboratory settings using cells or tissues. It is important to note that these products are not categorized as medicines or drugs, and they have not received approval from the FDA for the prevention, treatment, or cure of any medical condition, ailment, or disease. We must emphasize that any form of bodily introduction of these products into humans or animals is strictly prohibited by law. It is essential to adhere to these guidelines to ensure compliance with legal and ethical standards in research and experimentation.