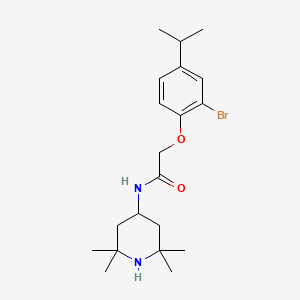
VU0134992
Overview
Description
VU 0134992 is a chemical compound known for its role as a selective blocker of the inward rectifier potassium channel Kir4.1. This compound has garnered significant attention in scientific research due to its specificity and effectiveness in modulating potassium channels, which are crucial for various physiological processes .
Mechanism of Action
Target of Action
VU0134992 is a subtype-preferring, orally active, and selective blocker of the Kir4.1 potassium channel . The Kir4.1 channel, encoded by the KCNJ10 gene, plays crucial physiological roles in epithelial cells of the kidney, astrocytes in the central nervous system, and stria vascularis of the inner ear .
Mode of Action
This compound interacts with the Kir4.1 channel, inhibiting its function. It has an IC50 value of 0.97 µM, indicating its potency in blocking the channel . It is 9-fold selective for homomeric Kir4.1 over Kir4.1/5.1 concatemeric channels at -120 mV .
Biochemical Pathways
The Kir4.1 channel is involved in the regulation of potassium (K+) transport and electrolyte homeostasis in various tissues . By blocking the Kir4.1 channel, this compound can disrupt these processes, leading to changes in cellular function and physiology.
Pharmacokinetics
This compound has been found to display a large free unbound fraction (fu) in rat plasma (fu = 0.213) . This suggests that a significant portion of the compound remains unbound to plasma proteins, potentially enhancing its bioavailability and distribution within the body.
Result of Action
The inhibition of the Kir4.1 channel by this compound has been associated with increased urinary sodium (Na+) and potassium (K+) excretion in rats . This indicates that the compound’s action can lead to diuretic, natriuretic, and kaliuretic effects.
Preparation Methods
Synthetic Routes and Reaction Conditions
The synthesis of VU 0134992 involves multiple steps, starting from commercially available precursors. The key steps typically include:
Formation of the core structure: This involves the construction of the central scaffold through a series of condensation and cyclization reactions.
Functionalization: Introduction of functional groups that are essential for the activity of the compound. This may involve halogenation, alkylation, or acylation reactions.
Purification: The final product is purified using techniques such as recrystallization or chromatography to achieve the desired purity.
Industrial Production Methods
While specific industrial production methods for VU 0134992 are not widely documented, the general approach would involve scaling up the laboratory synthesis process. This includes optimizing reaction conditions for larger batches, ensuring consistent quality, and implementing efficient purification techniques.
Chemical Reactions Analysis
Types of Reactions
VU 0134992 can undergo various chemical reactions, including:
Oxidation: This reaction involves the addition of oxygen or the removal of hydrogen, often using oxidizing agents like potassium permanganate or hydrogen peroxide.
Reduction: The addition of hydrogen or removal of oxygen, typically using reducing agents such as lithium aluminum hydride or sodium borohydride.
Substitution: Replacement of one functional group with another, which can be achieved using nucleophilic or electrophilic reagents.
Common Reagents and Conditions
Oxidation: Potassium permanganate, hydrogen peroxide.
Reduction: Lithium aluminum hydride, sodium borohydride.
Substitution: Halogenating agents, alkylating agents.
Major Products
The major products formed from these reactions depend on the specific conditions and reagents used. For instance, oxidation might yield hydroxylated derivatives, while reduction could produce deoxygenated compounds.
Scientific Research Applications
VU 0134992 has a wide range of applications in scientific research:
Chemistry: Used as a tool to study the properties and functions of potassium channels.
Biology: Helps in understanding the role of potassium channels in cellular physiology.
Medicine: Potential therapeutic applications in conditions related to potassium channel dysfunction, such as epilepsy and cardiac arrhythmias.
Industry: Utilized in the development of new pharmaceuticals and as a reference compound in drug discovery
Comparison with Similar Compounds
Similar Compounds
VU 0240551: Another Kir4.1 blocker with different selectivity and potency.
ML133: A blocker of Kir2.1 channels, used for comparative studies.
Tertiapin-Q: A peptide inhibitor of Kir1.1 and Kir3.1 channels.
Uniqueness
VU 0134992 is unique due to its high selectivity for the Kir4.1 channel over other potassium channels. This specificity makes it a valuable tool for studying the physiological and pathological roles of Kir4.1 without affecting other potassium channels .
Properties
IUPAC Name |
2-(2-bromo-4-propan-2-ylphenoxy)-N-(2,2,6,6-tetramethylpiperidin-4-yl)acetamide | |
---|---|---|
Source | PubChem | |
URL | https://pubchem.ncbi.nlm.nih.gov | |
Description | Data deposited in or computed by PubChem | |
InChI |
InChI=1S/C20H31BrN2O2/c1-13(2)14-7-8-17(16(21)9-14)25-12-18(24)22-15-10-19(3,4)23-20(5,6)11-15/h7-9,13,15,23H,10-12H2,1-6H3,(H,22,24) | |
Source | PubChem | |
URL | https://pubchem.ncbi.nlm.nih.gov | |
Description | Data deposited in or computed by PubChem | |
InChI Key |
OTTDLWFVHQYRDA-UHFFFAOYSA-N | |
Source | PubChem | |
URL | https://pubchem.ncbi.nlm.nih.gov | |
Description | Data deposited in or computed by PubChem | |
Canonical SMILES |
CC(C)C1=CC(=C(C=C1)OCC(=O)NC2CC(NC(C2)(C)C)(C)C)Br | |
Source | PubChem | |
URL | https://pubchem.ncbi.nlm.nih.gov | |
Description | Data deposited in or computed by PubChem | |
Molecular Formula |
C20H31BrN2O2 | |
Source | PubChem | |
URL | https://pubchem.ncbi.nlm.nih.gov | |
Description | Data deposited in or computed by PubChem | |
Molecular Weight |
411.4 g/mol | |
Source | PubChem | |
URL | https://pubchem.ncbi.nlm.nih.gov | |
Description | Data deposited in or computed by PubChem | |
Retrosynthesis Analysis
AI-Powered Synthesis Planning: Our tool employs the Template_relevance Pistachio, Template_relevance Bkms_metabolic, Template_relevance Pistachio_ringbreaker, Template_relevance Reaxys, Template_relevance Reaxys_biocatalysis model, leveraging a vast database of chemical reactions to predict feasible synthetic routes.
One-Step Synthesis Focus: Specifically designed for one-step synthesis, it provides concise and direct routes for your target compounds, streamlining the synthesis process.
Accurate Predictions: Utilizing the extensive PISTACHIO, BKMS_METABOLIC, PISTACHIO_RINGBREAKER, REAXYS, REAXYS_BIOCATALYSIS database, our tool offers high-accuracy predictions, reflecting the latest in chemical research and data.
Strategy Settings
Precursor scoring | Relevance Heuristic |
---|---|
Min. plausibility | 0.01 |
Model | Template_relevance |
Template Set | Pistachio/Bkms_metabolic/Pistachio_ringbreaker/Reaxys/Reaxys_biocatalysis |
Top-N result to add to graph | 6 |
Feasible Synthetic Routes
Q1: What is the mechanism of action of VU0134992?
A: this compound inhibits Kir4.1 channels by physically blocking the channel pore. [, , ] This blockage prevents potassium ions from flowing through the channel, disrupting the normal electrical activity of the cell. Specifically, this compound interacts with glutamate 158 and isoleucine 159, two critical residues lining the Kir4.1 channel pore. []
Q2: How does inhibiting Kir4.1 with this compound affect renal function?
A: Kir4.1 channels play a crucial role in potassium recycling and maintaining the negative membrane potential in renal cells, which is essential for sodium reabsorption. Inhibition of Kir4.1 by this compound disrupts this process, leading to decreased sodium reabsorption and consequently, diuresis (increased urine production), natriuresis (increased sodium excretion), and kaliuresis (increased potassium excretion). [, ]
Q3: What is the significance of this compound's effect on Kir4.1/5.1 heteromeric channels?
A: While this compound shows greater selectivity for Kir4.1 homomers, its ability to inhibit Kir4.1/5.1 heteromers is noteworthy. These heteromers are predominantly expressed in the distal convoluted tubule of the kidney and are involved in potassium sensing, blood pressure regulation, and sodium reabsorption via the sodium-chloride cotransporter NCC. [] Inhibition of Kir4.1/5.1 presents a potential therapeutic avenue for managing edema and hypertension. [, , ]
Q4: What are the implications of this compound for future research?
A: this compound represents a valuable tool compound for further investigating the physiological roles of Kir4.1 channels, particularly in the context of renal function and blood pressure control. Its diuretic, natriuretic, and kaliuretic effects suggest potential therapeutic applications for managing hypertension and edema, particularly in cases where loop diuretic resistance has developed. [, ] Furthermore, its selectivity profile and known mechanism of action provide a foundation for developing more potent and specific Kir4.1 inhibitors. []
- Discovery, Characterization, and Effects on Renal Fluid and Electrolyte Excretion of the Kir4.1 Potassium Channel Pore Blocker, this compound.
- Contribution of inwardly rectifying K+ channel 4.1 of supraoptic astrocytes to the regulation of vasopressin neuronal activity by hypotonicity.
- Development of Distal Nephron Diuretics Targeting Heteromeric Kir4.1/5.1 Potassium Channels.
- Next-generation inward rectifier potassium channel modulators: Discovery and molecular pharmacology.
- Role of Basolateral Kir4.1/Kir5.1 Channel in the Regulation of Electrolyte Balance and ENaC Activity in the Cortical Collecting Duct.
Disclaimer and Information on In-Vitro Research Products
Please be aware that all articles and product information presented on BenchChem are intended solely for informational purposes. The products available for purchase on BenchChem are specifically designed for in-vitro studies, which are conducted outside of living organisms. In-vitro studies, derived from the Latin term "in glass," involve experiments performed in controlled laboratory settings using cells or tissues. It is important to note that these products are not categorized as medicines or drugs, and they have not received approval from the FDA for the prevention, treatment, or cure of any medical condition, ailment, or disease. We must emphasize that any form of bodily introduction of these products into humans or animals is strictly prohibited by law. It is essential to adhere to these guidelines to ensure compliance with legal and ethical standards in research and experimentation.