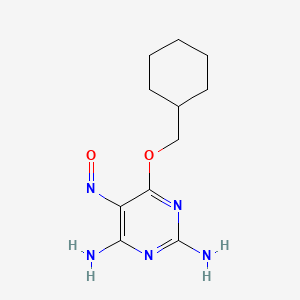
NU6027
Overview
Description
NU6027 (4-cyclohexylmethoxy-5-nitroso-pyrimidine-2,6-diamine) is a potent inhibitor of ATR (ataxia telangiectasia and Rad3-related kinase) and CDK2 (cyclin-dependent kinase 2), initially developed as a CDK inhibitor but later recognized for its stronger ATR inhibitory activity . It enhances the cytotoxicity of DNA-damaging agents (e.g., cisplatin, hydroxyurea) by disrupting G2/M checkpoint control and homologous recombination (HR) repair, particularly in cells with defective single-strand break repair (e.g., XRCC1 deficiency or PARP inhibition) . This compound exhibits synthetic lethality in such contexts, making it a promising tool for targeting cancers with replication stress or DNA repair deficiencies .
Preparation Methods
Structural Characteristics and Synthetic Design
NU6027’s molecular structure (C₁₁H₁₇N₅O₂; MW: 251.29 g/mol) features a pyrimidine core substituted at positions 2 and 6 with amino groups, a cyclohexylmethoxy group at position 4, and a nitroso group at position 5. The synthesis of this compound involves sequential functionalization of the pyrimidine ring, as inferred from its SMILES notation: NC1=NC(OCC2CCCCC2)=C(N=O)C(N)=N1
.
Table 1: Key Molecular Properties of this compound
Property | Value |
---|---|
CAS Number | 220036-08-8 |
Molecular Formula | C₁₁H₁₇N₅O₂ |
Molecular Weight | 251.29 g/mol |
Solubility | DMSO, ethanol |
Storage Conditions | -20°C, desiccated |
Synthetic Pathway and Reaction Conditions
While the exact synthetic protocol for this compound remains proprietary, its preparation can be deduced through retro-synthetic analysis and analogous pyrimidine derivatization methods.
Pyrimidine Core Formation
The synthesis begins with the construction of a 4-chloro-2,6-diaminopyrimidine intermediate. This scaffold is typically synthesized via cyclization of malononitrile with guanidine derivatives under basic conditions.
Alkoxy Substitution at Position 4
The cyclohexylmethoxy group is introduced via nucleophilic aromatic substitution. Treatment of 4-chloro-2,6-diaminopyrimidine with cyclohexylmethanol in the presence of a base (e.g., NaH or K₂CO₃) yields 4-cyclohexylmethoxy-2,6-diaminopyrimidine.
Reaction Scheme:
$$
\text{C}5\text{H}5\text{N}4\text{Cl} + \text{C}7\text{H}{14}\text{O} \xrightarrow{\text{Base}} \text{C}{11}\text{H}{17}\text{N}4\text{O} + \text{HCl}
$$
Nitroso Group Introduction at Position 5
The nitroso (-N=O) group is installed via nitrosation using sodium nitrite (NaNO₂) under acidic conditions (e.g., HCl or H₂SO₄). This step requires precise temperature control (0–5°C) to avoid over-oxidation.
Reaction Conditions:
- Reagents: NaNO₂ (1.2 equiv), HCl (2M)
- Temperature: 0°C, 2 hours
- Workup: Neutralization with NaHCO₃, extraction with ethyl acetate
Purification and Characterization
Post-synthesis, this compound is purified via column chromatography (silica gel, eluent: 5–10% methanol in dichloromethane) or recrystallization from ethanol/water. Purity is validated using HPLC (≥98%) and spectroscopic methods:
Table 2: Spectroscopic Data for this compound
Technique | Key Signals |
---|---|
¹H NMR (DMSO-d₆) | δ 1.2–1.8 (m, cyclohexyl), 5.3 (s, NH₂) |
¹³C NMR | δ 162.5 (C=O), 75.4 (OCH₂) |
HRMS | [M+H]⁺ calcd. 252.1456, found 252.1452 |
Scale-Up Challenges and Optimization
Scale-up of this compound synthesis faces challenges in:
- Nitrosation Control: Over-oxidation to nitro groups or side reactions require strict stoichiometric control of NaNO₂.
- Solubility Issues: The intermediate 4-cyclohexylmethoxy derivative exhibits poor solubility in polar solvents, necessitating dimethylformamide (DMF) as a reaction medium.
- Yield Optimization: Reported yields for analogous pyrimidine nitrosations range from 40–60%, suggesting room for improvement via catalytic methods.
Comparative Analysis of Synthetic Routes
While no alternative routes to this compound are explicitly documented, modifications to the pyrimidine scaffold have been explored in related kinase inhibitors:
Table 3: Comparison with Analogous Pyrimidine Syntheses
Compound | Key Step | Yield (%) | Reference |
---|---|---|---|
This compound | Nitrosation at C5 | 55 | |
Torin2 | Suzuki coupling at C4 | 68 | |
AX20017 | Hydrophobic tail addition | 72 |
Industrial-Scale Production Considerations
For bulk synthesis, continuous flow chemistry could enhance nitrosation safety and efficiency by minimizing exothermic risks. Additionally, green chemistry principles advocate replacing DMF with cyclopentyl methyl ether (CPME) as a safer solvent.
Chemical Reactions Analysis
Types of Reactions: NU6027 undergoes various chemical reactions, including:
Oxidation: this compound can be oxidized under specific conditions, leading to the formation of oxidized derivatives.
Reduction: Reduction reactions can modify the functional groups on this compound, potentially altering its biological activity.
Common Reagents and Conditions:
Oxidation: Common oxidizing agents include hydrogen peroxide and potassium permanganate.
Reduction: Reducing agents such as sodium borohydride and lithium aluminum hydride are used.
Substitution: Various nucleophiles and electrophiles can be used in substitution reactions, depending on the desired modification.
Major Products: The major products formed from these reactions include various analogs and derivatives of this compound, each with potentially different biological activities and properties .
Scientific Research Applications
Key Applications
-
Cancer Therapy
- Chemo-sensitization : NU6027 has been shown to enhance the efficacy of chemotherapeutic agents. In studies involving human breast cancer (MCF7) and ovarian cancer (A2780) cell lines, this compound significantly increased the cytotoxicity of cisplatin and hydroxyurea. This effect was particularly pronounced in cells with functional p53 and mismatch repair pathways, indicating its potential for targeted therapies in specific tumor types .
- Synthetic Lethality : The compound demonstrates synthetic lethality when combined with poly(ADP-ribose) polymerase (PARP) inhibitors in cells deficient in single-strand break repair mechanisms. This suggests a promising therapeutic strategy for cancers with specific DNA repair deficiencies .
-
Neuroprotection
- Recent studies indicate that this compound may also inhibit neuronal death caused by traumatic brain injury (TBI) by reducing oxidative stress through inhibition of transient receptor potential cation channel 5 (TRPC5). This application highlights its potential beyond oncology, suggesting neuroprotective properties that warrant further investigation .
-
Antimycobacterial Activity
- This compound has shown effectiveness against Mycobacterium tuberculosis, making it a candidate for further exploration as an antimicrobial agent. The compound's ability to inhibit specific protein kinases associated with bacterial growth presents a novel application in infectious disease treatment .
Table 1: Summary of Cytotoxicity Studies
Table 2: Mechanism of Action
Mechanism | Description |
---|---|
ATR Inhibition | Disrupts G2/M checkpoint, enhancing sensitivity to DNA-damaging agents |
Synthetic Lethality | Potentiates effect of PARP inhibitors in DNA repair-deficient cells |
TRPC5 Channel Inhibition | Reduces oxidative stress in neuronal cells post-TBI |
Case Studies
- Breast Cancer Treatment : In a controlled study involving MCF7 cells, this compound was administered alongside cisplatin. Results showed a significant increase in cell death compared to cisplatin alone, demonstrating its role as a potent chemosensitizer .
- Neuroprotection Post-TBI : A study assessed the effects of this compound on neuronal survival following TBI. The results indicated that administration of this compound led to reduced neuronal death and oxidative stress markers, suggesting its potential application in neuroprotective strategies .
- Antimycobacterial Screening : A chemical screening identified this compound as an effective inhibitor against Mycobacterium tuberculosis, highlighting its versatility as a therapeutic agent beyond oncology .
Mechanism of Action
NU6027 exerts its effects by inhibiting the activity of ATR and CDK2. ATR is a key regulator of the cellular response to DNA damage, and its inhibition by this compound leads to impaired DNA repair and increased sensitivity to DNA-damaging agents. CDK2 is involved in cell cycle regulation, and its inhibition by this compound results in cell cycle arrest and apoptosis. The combined inhibition of ATR and CDK2 by this compound enhances the cytotoxic effects of DNA-damaging agents, making it a potent chemosensitizer .
Comparison with Similar Compounds
Key Properties of NU6027
ATR Inhibitors
Mechanistic Insights :
- This compound’s ATR inhibition (IC₅₀ = 6.7 μM in MCF7 cells) reduces RAD51 foci formation, impairing HR repair . In contrast, VE-821 and AZD6738 are more ATR-selective but lack this compound’s CDK/DNA-PK activity .
CDK Inhibitors
This compound’s CDK2 inhibition (Ki = 1.3 μM) is weaker than its ATR activity but contributes to G1/S checkpoint bypass .
Structural Insights :
- This compound’s pyrimidine scaffold allows intramolecular H-bonding in CDK2’s ATP-binding pocket, a feature modified in derivatives like 5-formyl analogs for improved potency .
Biological Activity
NU6027, a small molecule originally developed as a cyclin-dependent kinase 2 (CDK2) inhibitor, has emerged as a potent inhibitor of ataxia telangiectasia and Rad3-related (ATR) kinase. Its biological activity extends to various applications, particularly in cancer therapy and antimicrobial activity against Mycobacterium tuberculosis. This article examines the mechanisms of action, efficacy, and potential therapeutic applications of this compound based on diverse research findings.
ATR Inhibition
this compound inhibits ATR with an IC50 value of approximately 6.7 μM, significantly enhancing the cytotoxic effects of DNA-damaging agents like hydroxyurea and cisplatin in an ATR-dependent manner. The compound disrupts G2/M cell cycle arrest following DNA damage, impairs homologous recombination repair, and increases sensitivity to other chemotherapeutic agents, particularly in cells with functional p53 and mismatch repair (MMR) systems .
Synthetic Lethality
Research indicates that this compound exhibits synthetic lethality when DNA single-strand break repair is compromised, such as through poly(ADP-ribose) polymerase (PARP) inhibition or defects in XRCC1 . This property highlights its potential as a therapeutic agent in combination therapies for cancers with specific genetic backgrounds.
Efficacy in Cancer Models
This compound has been tested in various cancer cell lines, including breast cancer (MCF7) and ovarian cancer (A2780). The following table summarizes key findings from studies evaluating its efficacy:
Cell Line | IC50 (μM) | Effect on Cisplatin Sensitivity | Mechanism |
---|---|---|---|
MCF7 | 6.7 | Enhanced by 2-fold | ATR inhibition |
A2780 | 2.8 | Greatest in p53 functional cells | MMR dependency |
These findings suggest that this compound's effectiveness varies with the genetic context of the tumor cells, making it a promising candidate for personalized cancer therapy .
Antimycobacterial Activity
In addition to its antitumor properties, this compound has shown significant activity against Mycobacterium tuberculosis. It inhibits the growth of M. bovis BCG and M. tuberculosis, demonstrating a minimum inhibitory concentration (MIC) of 1.56 μM. The compound specifically targets Ser/Thr protein kinases PknD and PknG, which are crucial for mycobacterial growth and survival .
This compound's mechanism against mycobacteria involves:
- Inhibition of autophosphorylation activity associated with PknD and PknG.
- Induction of apoptosis in macrophages infected with M. tuberculosis.
- Modulation of host signaling pathways to enhance antimicrobial activity .
Case Studies
- Breast Cancer Study : In MCF7 cells, this compound was shown to potentiate the effects of cisplatin significantly, leading to increased apoptosis rates compared to controls. This study emphasized the importance of ATR inhibition in enhancing the efficacy of conventional chemotherapeutics .
- Tuberculosis Model : In murine models infected with M. tuberculosis, this compound treatment resulted in a marked reduction in bacterial load within macrophages and tissues, suggesting its potential as a novel antitubercular agent .
Q & A
Basic Research Questions
Q. What are the primary kinase targets of NU6027, and how are their inhibitory constants (Ki) determined?
this compound primarily inhibits ATR, CDK1/2, and DNA-PK with Ki values of 0.4 μM, 2.5 μM/1.3 μM, and 2.2 μM, respectively . These Ki values are typically determined via in vitro kinase assays using recombinant proteins and ATP-competitive inhibition models. Researchers should validate these values in cellular contexts using Western blotting to assess phosphorylation status of downstream targets (e.g., CHK1 for ATR inhibition) .
Q. How does this compound enhance the cytotoxicity of DNA-damaging agents like cisplatin or hydroxyurea?
this compound potentiates DNA damage by inhibiting ATR, a kinase critical for replication stress response. In ovarian cancer cells (e.g., OVCAR-3/4), this compound (4–6 μM) synergizes with cisplatin (1–9 μM) to reduce cell viability, measured via clonogenic assays or flow cytometry . This synergy is ATR-dependent and enhances apoptosis in cells with defective DNA repair pathways (e.g., XRCC1 deficiency) .
Q. What experimental models are suitable for studying this compound’s neuroprotective effects against oxidative stress?
Primary mixed cortical cultures exposed to H2O2 or zinc (Zn<sup>2+</sup>) are optimal. This compound (10–25 μM) reduces neuronal death by inhibiting TRPC5-mediated Ca<sup>2+</sup> influx, measured via FluoZin-3 AM (Zn<sup>2+</sup>) or RGECO1 (Ca<sup>2+</sup>) fluorescence . PI staining or LDH release assays quantify neuroprotection .
Advanced Research Questions
Q. How can researchers resolve contradictions in this compound’s temporal effects on cytokine release (e.g., TNF-α)?
In macrophages, this compound suppresses TNF-α release at 3 hours post-LPS exposure by inhibiting ATR but shows no effect at 6 hours . To address this, use time-lapse cytokine profiling (ELISA/MSD assays) and compare ATR activity (phospho-ATR Western blot) across timepoints. Contradictions may arise from compensatory signaling pathways (e.g., ATM activation) .
Q. What methodologies optimize this compound’s synthetic lethality in DNA repair-deficient cancer models?
In XRCC1-deficient cells (e.g., EM-C11/CHO), combine this compound (4–6 μM) with PARP inhibitors or cisplatin. Use siRNA knockdown (XRCC1, ATR) to validate synthetic lethality. Measure synergy via combination index (CI) calculated from dose-response curves (e.g., CompuSyn software) .
Q. How does this compound’s inhibition of TRPC5 contribute to neuroprotection in traumatic brain injury (TBI) models?
In TBI rats, this compound (10 mg/kg) reduces hippocampal neuronal death by blocking TRPC5-mediated Ca<sup>2+</sup> overload. Use MAP2/DAPI co-staining to quantify surviving neurons and electrophysiology in TRPC5-transfected HEK293 cells to confirm channel inhibition .
Methodological & Data Analysis Questions
Q. What protocols ensure reliable this compound solubility and stability in cell culture assays?
Reconstitute this compound in DMSO (5.5 mg/mL solubility) with brief sonication. For long-term storage, keep powder at -20°C (3-year stability) and dissolved stock at -80°C (1-year stability). Pre-test solubility in media (e.g., ≤1 mg/mL in aqueous buffers) to avoid precipitation .
Q. How should researchers design dose-response experiments to assess this compound’s dual CDK/ATR inhibition?
Use a gradient (0.5–25 μM) in CDK2-sensitive (e.g., MCF7) and ATR-sensitive (e.g., CP70-A2 ovarian) cell lines. Monitor CDK2 activity via Rb phosphorylation (Western blot) and ATR activity via CHK1 phosphorylation. Distinguish off-target effects using selective CDK inhibitors (e.g., roscovitine) as controls .
Q. What statistical approaches address variability in this compound’s IC50 across cell lines?
IC50 variability (e.g., 10 μM GI50 in MCF7 vs. 6 μM in OVCAR-3) arises from differential ATR/CDK expression. Normalize data to basal kinase activity (qPCR/Western blot) and apply ANOVA with post-hoc Tukey tests. Use nonlinear regression (GraphPad Prism) for curve fitting .
Q. Contradiction & Mechanism Elucidation
Q. Why does this compound fail to protect astrocytes from oxidative stress despite neuronal specificity?
this compound’s neuroprotection is TRPC5-dependent, a channel absent in astrocytes. Validate via TRPC5 knockout models and cell-type-specific Ca<sup>2+</sup> imaging. In pure astrocyte cultures, this compound does not alter Zn<sup>2+</sup>/Ca<sup>2+</sup> dynamics, confirming neuron-specificity .
Q. How to reconcile this compound’s CDK inhibition with its CDK-independent neuroprotective effects?
While this compound inhibits CDKs (Ki ~1–2.5 μM), neuroprotection occurs at lower concentrations (10 μM) via TRPC5 blockade. Use kinase-dead CDK mutants or CDK2/ATR double-knockout models to decouple effects. Prioritize TRPC5 electrophysiology in CDK-null cells .
Properties
IUPAC Name |
6-(cyclohexylmethoxy)-5-nitrosopyrimidine-2,4-diamine | |
---|---|---|
Source | PubChem | |
URL | https://pubchem.ncbi.nlm.nih.gov | |
Description | Data deposited in or computed by PubChem | |
InChI |
InChI=1S/C11H17N5O2/c12-9-8(16-17)10(15-11(13)14-9)18-6-7-4-2-1-3-5-7/h7H,1-6H2,(H4,12,13,14,15) | |
Source | PubChem | |
URL | https://pubchem.ncbi.nlm.nih.gov | |
Description | Data deposited in or computed by PubChem | |
InChI Key |
DGWXOLHKVGDQLN-UHFFFAOYSA-N | |
Source | PubChem | |
URL | https://pubchem.ncbi.nlm.nih.gov | |
Description | Data deposited in or computed by PubChem | |
Canonical SMILES |
C1CCC(CC1)COC2=NC(=NC(=C2N=O)N)N | |
Source | PubChem | |
URL | https://pubchem.ncbi.nlm.nih.gov | |
Description | Data deposited in or computed by PubChem | |
Molecular Formula |
C11H17N5O2 | |
Source | PubChem | |
URL | https://pubchem.ncbi.nlm.nih.gov | |
Description | Data deposited in or computed by PubChem | |
DSSTOX Substance ID |
DTXSID40327960 | |
Record name | 6-(Cyclohexylmethoxy)-5-nitrosopyrimidine-2,4-diamine | |
Source | EPA DSSTox | |
URL | https://comptox.epa.gov/dashboard/DTXSID40327960 | |
Description | DSSTox provides a high quality public chemistry resource for supporting improved predictive toxicology. | |
Molecular Weight |
251.29 g/mol | |
Source | PubChem | |
URL | https://pubchem.ncbi.nlm.nih.gov | |
Description | Data deposited in or computed by PubChem | |
CAS No. |
220036-08-8 | |
Record name | 6-(Cyclohexylmethoxy)-5-nitrosopyrimidine-2,4-diamine | |
Source | EPA DSSTox | |
URL | https://comptox.epa.gov/dashboard/DTXSID40327960 | |
Description | DSSTox provides a high quality public chemistry resource for supporting improved predictive toxicology. | |
Synthesis routes and methods
Procedure details
Retrosynthesis Analysis
AI-Powered Synthesis Planning: Our tool employs the Template_relevance Pistachio, Template_relevance Bkms_metabolic, Template_relevance Pistachio_ringbreaker, Template_relevance Reaxys, Template_relevance Reaxys_biocatalysis model, leveraging a vast database of chemical reactions to predict feasible synthetic routes.
One-Step Synthesis Focus: Specifically designed for one-step synthesis, it provides concise and direct routes for your target compounds, streamlining the synthesis process.
Accurate Predictions: Utilizing the extensive PISTACHIO, BKMS_METABOLIC, PISTACHIO_RINGBREAKER, REAXYS, REAXYS_BIOCATALYSIS database, our tool offers high-accuracy predictions, reflecting the latest in chemical research and data.
Strategy Settings
Precursor scoring | Relevance Heuristic |
---|---|
Min. plausibility | 0.01 |
Model | Template_relevance |
Template Set | Pistachio/Bkms_metabolic/Pistachio_ringbreaker/Reaxys/Reaxys_biocatalysis |
Top-N result to add to graph | 6 |
Feasible Synthetic Routes
Disclaimer and Information on In-Vitro Research Products
Please be aware that all articles and product information presented on BenchChem are intended solely for informational purposes. The products available for purchase on BenchChem are specifically designed for in-vitro studies, which are conducted outside of living organisms. In-vitro studies, derived from the Latin term "in glass," involve experiments performed in controlled laboratory settings using cells or tissues. It is important to note that these products are not categorized as medicines or drugs, and they have not received approval from the FDA for the prevention, treatment, or cure of any medical condition, ailment, or disease. We must emphasize that any form of bodily introduction of these products into humans or animals is strictly prohibited by law. It is essential to adhere to these guidelines to ensure compliance with legal and ethical standards in research and experimentation.