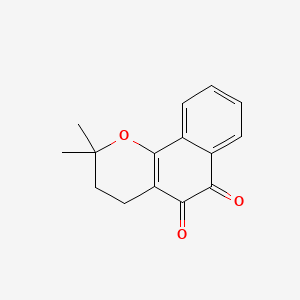
beta-Lapachone
Overview
Description
Beta-Lapachone is a benzochromenone that is 3,4-dihydro-2H-benzo [h]chromene-5,6-dione substituted by geminal methyl groups at position 2 . It is isolated from Tabebuia avellanedae and exhibits antineoplastic and anti-inflammatory activities . It has a role as an antineoplastic agent, an anti-inflammatory agent, and a plant metabolite .
Synthesis Analysis
Beta-Lapachone can be obtained through synthesis from lapachol, naphthoquinones, and other aromatic compounds . Two synthetic molecules (βLAP and NβL) and two inclusion complexes (ICβLAP and ICNβL) were tested at concentrations ranging from 1 μM to 500 μM .
Molecular Structure Analysis
The molecular formula of Beta-Lapachone is C15H14O3 . Its IUPAC name is 2,2-dimethyl-3,4-dihydrobenzo [h]chromene-5,6-dione . The molecular weight is 242.27 g/mol .
Chemical Reactions Analysis
Beta-Lapachone’s therapeutic efficacy partially stems from the drug’s induction of a futile NQO1-mediated redox cycle that causes high levels of superoxide and then peroxide formation, which damages DNA . It also has the possibility of isomerization in the human body .
Physical And Chemical Properties Analysis
Beta-Lapachone is a natural product found in Catalpa longissima, Handroanthus guayacan, and other organisms with data available . Numerous derivatives of Beta-Lapachone have been reported with aims to generate new chemical entities, improve the corresponding biological potency, and overcome disadvantages of its physical and chemical properties and safety profiles .
Scientific Research Applications
Cancer Treatment
Beta-Lapachone: is known for its anticancer properties, particularly due to its activation by the enzyme NAD(P)H:quinone oxidoreductase (NQO1), which is over-expressed in many tumors. It has shown antiproliferative activity against cancer cell lines and promotes immunogenic cell death in tumors .
Drug Solubilization
Due to its poor solubility in water, various formulation approaches like micellar systems, liposomes, and cyclodextrins have been proposed to enhance the solubility of beta-Lapachone for therapeutic applications .
Enzyme Inhibition
Beta-Lapachone: has been observed to inhibit topoisomerase I, an enzyme involved in DNA replication. This inhibition is another mechanism through which it exerts its anticancer effects .
Oxidative Stress Induction
This compound can induce oxidative stress selectively in cancer cells by disrupting their redox balance, which leads to cell death without affecting normal cells .
Immunomodulation
Research suggests that beta-Lapachone may influence the immune system by affecting tumor-associated neutrophils, potentially altering the tumor microenvironment and aiding in cancer treatment .
Molecular Targeting
It has been found to downregulate various molecular targets such as p53, TrkA, p38 MAPK, and others in oxaliplatin-resistant colorectal cancer cells, showcasing its potential as a targeted anticancer agent .
Mechanism of Action
Target of Action
Beta-Lapachone (β-Lapachone) primarily targets NAD(P)H:quinone oxidoreductase 1 (NQO1) . NQO1 is overexpressed in most solid cancers, making it a promising target for tumor selective killing .
Mode of Action
Beta-Lapachone, an o-naphthoquinone, induces a novel caspase- and p53-independent apoptotic pathway dependent on NQO1 . NQO1 reduces beta-Lapachone to an unstable hydroquinone that rapidly undergoes a two-step oxidation back to the parent compound, perpetuating a futile redox cycle .
Biochemical Pathways
Beta-Lapachone modulates cellular NAD+/NADH ratio, which has been associated with aging and anti-aging mechanisms of calorie restriction . It also induces immunogenic cell death in the tumor microenvironment (TME) and synergizes with anti-PD-L1 immunotherapy .
Pharmacokinetics
Its low oral bioavailability is mainly due to its extensive first-pass metabolic degradation as well as its low aqueous solubility and slow dissolution rate on intestinal lumen . New formulations and strategies have been developed to increase the solubility and to improve pharmacokinetics parameters for both oral and parenteral administration .
Result of Action
Beta-Lapachone exhibits significant antitumor effects on NQO1+ human solid cancer cell lines . It also induces DNA damage and nuclear abnormalities, such as nucleoplasmic bridges and nuclear buds . It also causes harmful effects in these cells, suggesting that the use of beta-lapachone in treating cancer should be carried out with caution .
Action Environment
The tumor microenvironment (TME) plays a significant role in the action of beta-Lapachone . The compound promotes tumor-associated neutrophils (TANs) polarized towards an anti-tumor (N1) phenotype . Depletion of neutrophils by co-addition of anti-Ly6G antibody abolished beta-Lapachone-induced anti-tumor efficacy in immune competent mice . Furthermore, TLR/MyD88 signaling deficiency decreased beta-Lapachone induced neutrophil tumor infiltration .
Safety and Hazards
Future Directions
NQO1 could be a useful prognostic biomarker for patients with breast cancer, and its bioactivatable drug, Beta-Lapachone, represents a promising new development and an effective strategy for indicating the progression of NQO1-positive breast cancers . Extensive efforts are required to explore novel therapeutic targets to control the invasion and metastasis of breast cancer and to improve the quality of life among breast cancer patients .
properties
IUPAC Name |
2,2-dimethyl-3,4-dihydrobenzo[h]chromene-5,6-dione | |
---|---|---|
Source | PubChem | |
URL | https://pubchem.ncbi.nlm.nih.gov | |
Description | Data deposited in or computed by PubChem | |
InChI |
InChI=1S/C15H14O3/c1-15(2)8-7-11-13(17)12(16)9-5-3-4-6-10(9)14(11)18-15/h3-6H,7-8H2,1-2H3 | |
Source | PubChem | |
URL | https://pubchem.ncbi.nlm.nih.gov | |
Description | Data deposited in or computed by PubChem | |
InChI Key |
QZPQTZZNNJUOLS-UHFFFAOYSA-N | |
Source | PubChem | |
URL | https://pubchem.ncbi.nlm.nih.gov | |
Description | Data deposited in or computed by PubChem | |
Canonical SMILES |
CC1(CCC2=C(O1)C3=CC=CC=C3C(=O)C2=O)C | |
Source | PubChem | |
URL | https://pubchem.ncbi.nlm.nih.gov | |
Description | Data deposited in or computed by PubChem | |
Molecular Formula |
C15H14O3 | |
Source | PubChem | |
URL | https://pubchem.ncbi.nlm.nih.gov | |
Description | Data deposited in or computed by PubChem | |
DSSTOX Substance ID |
DTXSID90197019 | |
Record name | beta-Lapachone | |
Source | EPA DSSTox | |
URL | https://comptox.epa.gov/dashboard/DTXSID90197019 | |
Description | DSSTox provides a high quality public chemistry resource for supporting improved predictive toxicology. | |
Molecular Weight |
242.27 g/mol | |
Source | PubChem | |
URL | https://pubchem.ncbi.nlm.nih.gov | |
Description | Data deposited in or computed by PubChem | |
Product Name |
beta-Lapachone | |
CAS RN |
4707-32-8 | |
Record name | β-Lapachone | |
Source | CAS Common Chemistry | |
URL | https://commonchemistry.cas.org/detail?cas_rn=4707-32-8 | |
Description | CAS Common Chemistry is an open community resource for accessing chemical information. Nearly 500,000 chemical substances from CAS REGISTRY cover areas of community interest, including common and frequently regulated chemicals, and those relevant to high school and undergraduate chemistry classes. This chemical information, curated by our expert scientists, is provided in alignment with our mission as a division of the American Chemical Society. | |
Explanation | The data from CAS Common Chemistry is provided under a CC-BY-NC 4.0 license, unless otherwise stated. | |
Record name | beta-Lapachone | |
Source | ChemIDplus | |
URL | https://pubchem.ncbi.nlm.nih.gov/substance/?source=chemidplus&sourceid=0004707328 | |
Description | ChemIDplus is a free, web search system that provides access to the structure and nomenclature authority files used for the identification of chemical substances cited in National Library of Medicine (NLM) databases, including the TOXNET system. | |
Record name | Lapachone | |
Source | DrugBank | |
URL | https://www.drugbank.ca/drugs/DB11948 | |
Description | The DrugBank database is a unique bioinformatics and cheminformatics resource that combines detailed drug (i.e. chemical, pharmacological and pharmaceutical) data with comprehensive drug target (i.e. sequence, structure, and pathway) information. | |
Explanation | Creative Common's Attribution-NonCommercial 4.0 International License (http://creativecommons.org/licenses/by-nc/4.0/legalcode) | |
Record name | 4707-32-8 | |
Source | DTP/NCI | |
URL | https://dtp.cancer.gov/dtpstandard/servlet/dwindex?searchtype=NSC&outputformat=html&searchlist=26326 | |
Description | The NCI Development Therapeutics Program (DTP) provides services and resources to the academic and private-sector research communities worldwide to facilitate the discovery and development of new cancer therapeutic agents. | |
Explanation | Unless otherwise indicated, all text within NCI products is free of copyright and may be reused without our permission. Credit the National Cancer Institute as the source. | |
Record name | beta-Lapachone | |
Source | EPA DSSTox | |
URL | https://comptox.epa.gov/dashboard/DTXSID90197019 | |
Description | DSSTox provides a high quality public chemistry resource for supporting improved predictive toxicology. | |
Record name | LAPACHONE | |
Source | FDA Global Substance Registration System (GSRS) | |
URL | https://gsrs.ncats.nih.gov/ginas/app/beta/substances/6N4FA2QQ6A | |
Description | The FDA Global Substance Registration System (GSRS) enables the efficient and accurate exchange of information on what substances are in regulated products. Instead of relying on names, which vary across regulatory domains, countries, and regions, the GSRS knowledge base makes it possible for substances to be defined by standardized, scientific descriptions. | |
Explanation | Unless otherwise noted, the contents of the FDA website (www.fda.gov), both text and graphics, are not copyrighted. They are in the public domain and may be republished, reprinted and otherwise used freely by anyone without the need to obtain permission from FDA. Credit to the U.S. Food and Drug Administration as the source is appreciated but not required. | |
Synthesis routes and methods I
Procedure details
Synthesis routes and methods II
Procedure details
Synthesis routes and methods III
Procedure details
Retrosynthesis Analysis
AI-Powered Synthesis Planning: Our tool employs the Template_relevance Pistachio, Template_relevance Bkms_metabolic, Template_relevance Pistachio_ringbreaker, Template_relevance Reaxys, Template_relevance Reaxys_biocatalysis model, leveraging a vast database of chemical reactions to predict feasible synthetic routes.
One-Step Synthesis Focus: Specifically designed for one-step synthesis, it provides concise and direct routes for your target compounds, streamlining the synthesis process.
Accurate Predictions: Utilizing the extensive PISTACHIO, BKMS_METABOLIC, PISTACHIO_RINGBREAKER, REAXYS, REAXYS_BIOCATALYSIS database, our tool offers high-accuracy predictions, reflecting the latest in chemical research and data.
Strategy Settings
Precursor scoring | Relevance Heuristic |
---|---|
Min. plausibility | 0.01 |
Model | Template_relevance |
Template Set | Pistachio/Bkms_metabolic/Pistachio_ringbreaker/Reaxys/Reaxys_biocatalysis |
Top-N result to add to graph | 6 |
Feasible Synthetic Routes
Disclaimer and Information on In-Vitro Research Products
Please be aware that all articles and product information presented on BenchChem are intended solely for informational purposes. The products available for purchase on BenchChem are specifically designed for in-vitro studies, which are conducted outside of living organisms. In-vitro studies, derived from the Latin term "in glass," involve experiments performed in controlled laboratory settings using cells or tissues. It is important to note that these products are not categorized as medicines or drugs, and they have not received approval from the FDA for the prevention, treatment, or cure of any medical condition, ailment, or disease. We must emphasize that any form of bodily introduction of these products into humans or animals is strictly prohibited by law. It is essential to adhere to these guidelines to ensure compliance with legal and ethical standards in research and experimentation.