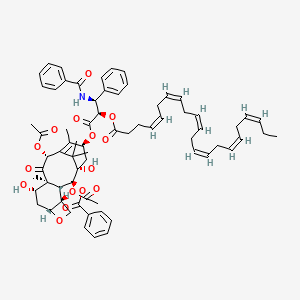
DHA-paclitaxel
Overview
Description
Docosahexaenoic acid-paclitaxel is a novel compound that combines the natural fatty acid docosahexaenoic acid with the well-known anticancer drug paclitaxel. This conjugation aims to enhance the delivery and efficacy of paclitaxel by leveraging the properties of docosahexaenoic acid, which is easily taken up by tumor cells . Docosahexaenoic acid-paclitaxel is designed to improve the pharmacokinetic profile and reduce the toxicity associated with traditional paclitaxel formulations .
Mechanism of Action
Target of Action
DHA-Paclitaxel, also known as Taxoprexin, primarily targets microtubules in the cell . It also interacts with the apoptosis regulator Bcl-2 .
Mode of Action
This compound is a prodrug composed of the natural fatty acid docosahexaenoic acid (DHA) covalently conjugated to the anti-microtubule agent paclitaxel . The paclitaxel moiety binds to tubulin and inhibits the disassembly of microtubules, thereby resulting in the inhibition of cell division . Paclitaxel also induces apoptosis by binding to and blocking the function of the apoptosis inhibitor protein Bcl-2 .
Biochemical Pathways
The action of this compound leads to the stabilization of microtubules, cell arrest, and subsequent apoptosis . It also inhibits the TLR4 signaling pathway, increases the immunomodulatory effects of the drug, and activates ER stress-mediated cell death in different cancers .
Pharmacokinetics
This compound exhibits improved pharmacokinetic and toxicity profiles when compared to conventional paclitaxel . It has a prolonged half-life and sustained levels within the plasma . The drug is extensively bound to human plasma . The fraction of unbound paclitaxel increases with an increase in this compound concentration, suggesting weakly competitive drug displacement from protein-binding sites . The area under the curve of unbound paclitaxel increases nonlinearly with dose .
Result of Action
The result of this compound’s action is the inhibition of cell division and the induction of apoptosis . This impedes the replication of tumor cells . The drug has demonstrated antineoplastic activity in animal models of cancer .
Action Environment
Environmental factors such as the presence of certain chemicals and types of electromagnetic radiation in the diet, environment, or workplace can influence the action, efficacy, and stability of this compound . In preclinical animal studies, DHA has been found to be preferentially taken up by tumor cells from the arterial blood supply, presumably for use as a precursor for metabolic and biochemical pathways .
Biochemical Analysis
Biochemical Properties
DHA-paclitaxel is a prodrug, meaning it is inactive until metabolized into its active components within the body . The compound is taken up by tumor cells, where the bond with DHA is cleaved, releasing the active paclitaxel . The released paclitaxel then binds to tubulin, a protein involved in cell division, and inhibits the disassembly of microtubules . This action disrupts cell division and leads to cell death .
Cellular Effects
This compound has been shown to have a significant impact on various types of cells and cellular processes. It is particularly effective against tumor cells, as these cells actively take up DHA . Once inside the cell, the this compound bond is cleaved, releasing paclitaxel which then exerts its cytotoxic effects . This compound has also been shown to induce apoptosis, or programmed cell death, by binding to and blocking the function of the apoptosis inhibitor protein Bcl-2 .
Molecular Mechanism
The molecular mechanism of this compound involves its conversion into its active components within the cell. The DHA component of the compound is taken up by tumor cells, where it is then cleaved to release paclitaxel . Paclitaxel then binds to tubulin, inhibiting the disassembly of microtubules . This disruption of the cell’s cytoskeleton interferes with cell division, leading to cell death . Additionally, paclitaxel induces apoptosis by binding to and blocking the function of the apoptosis inhibitor protein Bcl-2 .
Temporal Effects in Laboratory Settings
In laboratory settings, this compound has been shown to bind extensively to human plasma, indicating a nonspecific, nonsaturable process . This extensive binding to plasma proteins may explain the unique pharmacokinetic profile of this compound, which is characterized by a small volume of distribution and slow systemic clearance .
Dosage Effects in Animal Models
In animal models, this compound has demonstrated antineoplastic activity . The compound exhibits improved pharmacokinetic and toxicity profiles when compared to conventional paclitaxel
Metabolic Pathways
The metabolic pathways of this compound involve its uptake by tumor cells and subsequent cleavage to release the active paclitaxel
Transport and Distribution
This compound is transported and distributed within cells and tissues via its uptake by tumor cells . The compound’s ability to be taken up by tumor cells allows for targeted delivery of the active paclitaxel to these cells
Subcellular Localization
Given that the compound is taken up by tumor cells and cleaved to release paclitaxel , it is likely that this compound localizes to the same subcellular compartments as paclitaxel
Preparation Methods
Synthetic Routes and Reaction Conditions: The synthesis of docosahexaenoic acid-paclitaxel involves the covalent attachment of docosahexaenoic acid to the C2′-position of paclitaxel. This process typically includes the activation of docosahexaenoic acid followed by its conjugation to paclitaxel under specific reaction conditions . The reaction conditions often involve the use of solvents like acetonitrile and catalysts to facilitate the conjugation .
Industrial Production Methods: Industrial production of docosahexaenoic acid-paclitaxel follows similar synthetic routes but on a larger scale. The process is optimized for yield and purity, ensuring that the final product meets pharmaceutical standards. Techniques such as chromatography are employed to purify the compound .
Chemical Reactions Analysis
Types of Reactions: Docosahexaenoic acid-paclitaxel undergoes various chemical reactions, including hydrolysis and enzymatic cleavage. These reactions are crucial for releasing paclitaxel from the conjugate within the tumor cells .
Common Reagents and Conditions: The hydrolysis of docosahexaenoic acid-paclitaxel typically requires aqueous conditions and specific enzymes that target the ester bond between docosahexaenoic acid and paclitaxel .
Major Products: The primary product of these reactions is paclitaxel, which retains its pharmacological activity once released from the conjugate .
Scientific Research Applications
Docosahexaenoic acid-paclitaxel has a wide range of scientific research applications, particularly in the fields of chemistry, biology, medicine, and industry.
Chemistry: In chemistry, docosahexaenoic acid-paclitaxel serves as a model compound for studying drug conjugation and release mechanisms .
Biology: In biological research, it is used to investigate the cellular uptake and metabolism of fatty acid-drug conjugates .
Medicine: In medicine, docosahexaenoic acid-paclitaxel is primarily explored for its anticancer properties. It has shown promise in preclinical and clinical studies for treating various cancers, including breast, lung, and pancreatic cancers .
Industry: In the pharmaceutical industry, docosahexaenoic acid-paclitaxel is being developed as a potential therapeutic agent with improved efficacy and reduced side effects compared to traditional paclitaxel formulations .
Comparison with Similar Compounds
Docetaxel: Another taxane derivative with similar anticancer properties but different pharmacokinetic profiles.
DHA-SB-T-1214: A docosahexaenoic acid conjugate with a different taxane derivative, showing significant antitumor effects in preclinical studies.
Uniqueness: Docosahexaenoic acid-paclitaxel stands out due to its improved pharmacokinetic profile, reduced toxicity, and enhanced delivery to tumor cells compared to traditional paclitaxel and other derivatives .
Properties
IUPAC Name |
[(1S,2S,3R,4S,7R,9S,10S,12R,15S)-4,12-diacetyloxy-15-[(2R,3S)-3-benzamido-2-[(4Z,7Z,10Z,13Z,16Z,19Z)-docosa-4,7,10,13,16,19-hexaenoyl]oxy-3-phenylpropanoyl]oxy-1,9-dihydroxy-10,14,17,17-tetramethyl-11-oxo-6-oxatetracyclo[11.3.1.03,10.04,7]heptadec-13-en-2-yl] benzoate | |
---|---|---|
Source | PubChem | |
URL | https://pubchem.ncbi.nlm.nih.gov | |
Description | Data deposited in or computed by PubChem | |
InChI |
InChI=1S/C69H81NO15/c1-8-9-10-11-12-13-14-15-16-17-18-19-20-21-22-23-24-25-35-42-55(74)83-59(57(49-36-29-26-30-37-49)70-63(76)50-38-31-27-32-39-50)65(78)82-52-44-69(79)62(84-64(77)51-40-33-28-34-41-51)60-67(7,53(73)43-54-68(60,45-80-54)85-48(4)72)61(75)58(81-47(3)71)56(46(52)2)66(69,5)6/h9-10,12-13,15-16,18-19,21-22,24-34,36-41,52-54,57-60,62,73,79H,8,11,14,17,20,23,35,42-45H2,1-7H3,(H,70,76)/b10-9-,13-12-,16-15-,19-18-,22-21-,25-24-/t52-,53-,54+,57-,58+,59+,60-,62-,67+,68-,69+/m0/s1 | |
Source | PubChem | |
URL | https://pubchem.ncbi.nlm.nih.gov | |
Description | Data deposited in or computed by PubChem | |
InChI Key |
LRCZQSDQZJBHAF-PUBGEWHCSA-N | |
Source | PubChem | |
URL | https://pubchem.ncbi.nlm.nih.gov | |
Description | Data deposited in or computed by PubChem | |
Canonical SMILES |
CCC=CCC=CCC=CCC=CCC=CCC=CCCC(=O)OC(C(C1=CC=CC=C1)NC(=O)C2=CC=CC=C2)C(=O)OC3CC4(C(C5C(C(CC6C5(CO6)OC(=O)C)O)(C(=O)C(C(=C3C)C4(C)C)OC(=O)C)C)OC(=O)C7=CC=CC=C7)O | |
Source | PubChem | |
URL | https://pubchem.ncbi.nlm.nih.gov | |
Description | Data deposited in or computed by PubChem | |
Isomeric SMILES |
CC/C=C\C/C=C\C/C=C\C/C=C\C/C=C\C/C=C\CCC(=O)O[C@H]([C@H](C1=CC=CC=C1)NC(=O)C2=CC=CC=C2)C(=O)O[C@H]3C[C@]4([C@H]([C@H]5[C@@]([C@H](C[C@@H]6[C@]5(CO6)OC(=O)C)O)(C(=O)[C@@H](C(=C3C)C4(C)C)OC(=O)C)C)OC(=O)C7=CC=CC=C7)O | |
Source | PubChem | |
URL | https://pubchem.ncbi.nlm.nih.gov | |
Description | Data deposited in or computed by PubChem | |
Molecular Formula |
C69H81NO15 | |
Source | PubChem | |
URL | https://pubchem.ncbi.nlm.nih.gov | |
Description | Data deposited in or computed by PubChem | |
DSSTOX Substance ID |
DTXSID00873216 | |
Record name | DHA-Paclitaxel | |
Source | EPA DSSTox | |
URL | https://comptox.epa.gov/dashboard/DTXSID00873216 | |
Description | DSSTox provides a high quality public chemistry resource for supporting improved predictive toxicology. | |
Molecular Weight |
1164.4 g/mol | |
Source | PubChem | |
URL | https://pubchem.ncbi.nlm.nih.gov | |
Description | Data deposited in or computed by PubChem | |
Mechanism of Action |
A prodrug comprised of the naturally occurring omega-3 fatty acid docosahexaenoic acid (DHA) covalently conjugated to the anti-microtubule agent paclitaxel. Because tumor cells take up DHA, DHA-paclitaxel is delivered directly to tumor tissue, where the paclitaxel moiety binds to tubulin and inhibits the disassembly of microtubules, thereby resulting in the inhibition of cell division. Paclitaxel also induces apoptosis by binding to and blocking the function of the apoptosis inhibitor protein Bcl-2 (B-cell Leukemia 2). DHA-paclitaxel exhibits improved pharmacokinetic and toxicity profiles when compared to conventional paclitaxel and has demonstrated antineoplastic activity in animal models of cancer. | |
Record name | Paclitaxel docosahexaenoic acid | |
Source | DrugBank | |
URL | https://www.drugbank.ca/drugs/DB05297 | |
Description | The DrugBank database is a unique bioinformatics and cheminformatics resource that combines detailed drug (i.e. chemical, pharmacological and pharmaceutical) data with comprehensive drug target (i.e. sequence, structure, and pathway) information. | |
Explanation | Creative Common's Attribution-NonCommercial 4.0 International License (http://creativecommons.org/licenses/by-nc/4.0/legalcode) | |
CAS No. |
199796-52-6 | |
Record name | Taxoprexin | |
Source | CAS Common Chemistry | |
URL | https://commonchemistry.cas.org/detail?cas_rn=199796-52-6 | |
Description | CAS Common Chemistry is an open community resource for accessing chemical information. Nearly 500,000 chemical substances from CAS REGISTRY cover areas of community interest, including common and frequently regulated chemicals, and those relevant to high school and undergraduate chemistry classes. This chemical information, curated by our expert scientists, is provided in alignment with our mission as a division of the American Chemical Society. | |
Explanation | The data from CAS Common Chemistry is provided under a CC-BY-NC 4.0 license, unless otherwise stated. | |
Record name | Docosahexaenoyl-paclitaxel | |
Source | ChemIDplus | |
URL | https://pubchem.ncbi.nlm.nih.gov/substance/?source=chemidplus&sourceid=0199796526 | |
Description | ChemIDplus is a free, web search system that provides access to the structure and nomenclature authority files used for the identification of chemical substances cited in National Library of Medicine (NLM) databases, including the TOXNET system. | |
Record name | Paclitaxel docosahexaenoic acid | |
Source | DrugBank | |
URL | https://www.drugbank.ca/drugs/DB05297 | |
Description | The DrugBank database is a unique bioinformatics and cheminformatics resource that combines detailed drug (i.e. chemical, pharmacological and pharmaceutical) data with comprehensive drug target (i.e. sequence, structure, and pathway) information. | |
Explanation | Creative Common's Attribution-NonCommercial 4.0 International License (http://creativecommons.org/licenses/by-nc/4.0/legalcode) | |
Record name | DHA-Paclitaxel | |
Source | EPA DSSTox | |
URL | https://comptox.epa.gov/dashboard/DTXSID00873216 | |
Description | DSSTox provides a high quality public chemistry resource for supporting improved predictive toxicology. | |
Record name | PACLITAXEL DOCOSAHEXAENOIC ACID | |
Source | FDA Global Substance Registration System (GSRS) | |
URL | https://gsrs.ncats.nih.gov/ginas/app/beta/substances/OJE5810C4F | |
Description | The FDA Global Substance Registration System (GSRS) enables the efficient and accurate exchange of information on what substances are in regulated products. Instead of relying on names, which vary across regulatory domains, countries, and regions, the GSRS knowledge base makes it possible for substances to be defined by standardized, scientific descriptions. | |
Explanation | Unless otherwise noted, the contents of the FDA website (www.fda.gov), both text and graphics, are not copyrighted. They are in the public domain and may be republished, reprinted and otherwise used freely by anyone without the need to obtain permission from FDA. Credit to the U.S. Food and Drug Administration as the source is appreciated but not required. | |
Retrosynthesis Analysis
AI-Powered Synthesis Planning: Our tool employs the Template_relevance Pistachio, Template_relevance Bkms_metabolic, Template_relevance Pistachio_ringbreaker, Template_relevance Reaxys, Template_relevance Reaxys_biocatalysis model, leveraging a vast database of chemical reactions to predict feasible synthetic routes.
One-Step Synthesis Focus: Specifically designed for one-step synthesis, it provides concise and direct routes for your target compounds, streamlining the synthesis process.
Accurate Predictions: Utilizing the extensive PISTACHIO, BKMS_METABOLIC, PISTACHIO_RINGBREAKER, REAXYS, REAXYS_BIOCATALYSIS database, our tool offers high-accuracy predictions, reflecting the latest in chemical research and data.
Strategy Settings
Precursor scoring | Relevance Heuristic |
---|---|
Min. plausibility | 0.01 |
Model | Template_relevance |
Template Set | Pistachio/Bkms_metabolic/Pistachio_ringbreaker/Reaxys/Reaxys_biocatalysis |
Top-N result to add to graph | 6 |
Feasible Synthetic Routes
Disclaimer and Information on In-Vitro Research Products
Please be aware that all articles and product information presented on BenchChem are intended solely for informational purposes. The products available for purchase on BenchChem are specifically designed for in-vitro studies, which are conducted outside of living organisms. In-vitro studies, derived from the Latin term "in glass," involve experiments performed in controlled laboratory settings using cells or tissues. It is important to note that these products are not categorized as medicines or drugs, and they have not received approval from the FDA for the prevention, treatment, or cure of any medical condition, ailment, or disease. We must emphasize that any form of bodily introduction of these products into humans or animals is strictly prohibited by law. It is essential to adhere to these guidelines to ensure compliance with legal and ethical standards in research and experimentation.