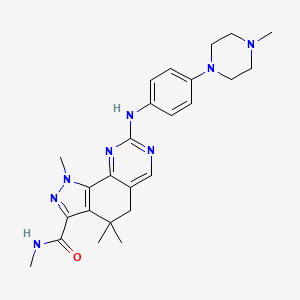
Milciclib
Overview
Description
Milciclib Maleate is a potent, small molecule inhibitor of multiple cyclin-dependent kinases, tropomyosin receptor kinases, and Src family kinases. It is primarily investigated for its potential antineoplastic activity, meaning it can inhibit the growth of tumors. This compound Maleate has shown promising results in clinical trials for various types of cancer, including thymic carcinoma and hepatocellular carcinoma .
Scientific Research Applications
Milciclib Maleate has a wide range of scientific research applications, particularly in the fields of chemistry, biology, medicine, and industry:
Chemistry: It is used as a tool compound to study the inhibition of cyclin-dependent kinases and other kinases.
Biology: It helps in understanding the role of kinases in cell cycle regulation and cancer progression.
Medicine: this compound Maleate is being investigated as a potential treatment for various cancers, including thymic carcinoma, hepatocellular carcinoma, and non-small cell lung cancer. .
Industry: It is used in the development of new cancer therapies and as a reference compound in drug discovery.
Mechanism of Action
Milciclib Maleate exerts its effects by inhibiting multiple cyclin-dependent kinases, tropomyosin receptor kinases, and Src family kinases. These kinases play crucial roles in cell cycle regulation, cell growth, and malignant progression of cancer. By inhibiting these kinases, this compound Maleate can suppress tumor growth and proliferation. The molecular targets and pathways involved include the inhibition of cyclin-dependent kinase 1, cyclin-dependent kinase 2, and cyclin-dependent kinase 4 .
Future Directions
Milciclib has shown promising results in clinical trials, particularly in combination with gemcitabine for treating non-small cell lung cancer (NSCLC) subjects with pan KRAS-positive mutations . The results warrant further clinical development .
Relevant Papers Several papers have been published on this compound. One paper discusses the association of PAFAH1B3 with poor prognosis and T-cell exhaustion microenvironment in hepatocellular carcinoma . Another paper discusses a phase I trial that evaluated the safety and tolerability of this compound in combination with gemcitabine in patients with refractory solid tumors .
Biochemical Analysis
Biochemical Properties
Milciclib is characterized as a potent nanomolar inhibitor of multiple cyclin-dependent kinases . It interacts with these enzymes, inhibiting their activity and thereby controlling cell growth . The nature of these interactions involves the binding of this compound to the active sites of these enzymes, preventing them from catalyzing their respective reactions .
Cellular Effects
This compound has demonstrated significant effects on various types of cells and cellular processes. It influences cell function by impacting cell signaling pathways, gene expression, and cellular metabolism . For instance, this compound has been shown to block glucose consumption in certain lung cancer cells by decreasing SLC2A1 (GLUT1) mRNA and protein levels and inhibiting glucose transport .
Molecular Mechanism
The mechanism of action of this compound involves its binding interactions with biomolecules, enzyme inhibition or activation, and changes in gene expression . This compound exerts its effects at the molecular level by binding to cyclin-dependent kinases and inhibiting their activity . This results in the downregulation of several proteins and pathways, providing a molecular basis for its therapeutic effects .
Temporal Effects in Laboratory Settings
In laboratory settings, the effects of this compound have been observed to change over time. In a Phase 2a trial, this compound was administered as a single therapy in sorafenib-resistant patients with hepatocellular carcinoma (HCC). The treatment was well-tolerated with manageable toxicities, and no drug-related deaths were recorded .
Dosage Effects in Animal Models
The effects of this compound have been studied in animal models, with the observed effects varying with different dosages . In an orthotopic model of HCC, sub-optimal doses of this compound were used to assess the synergistic or additive effects of the combination treatment .
Metabolic Pathways
This compound is involved in several metabolic pathways. It interacts with enzymes and cofactors within these pathways, influencing metabolic flux and metabolite levels . For instance, this compound has been shown to suppress the multiple tumorigenic pathways that are activated due to the activation of the KRAS gene .
Preparation Methods
The synthesis of Milciclib Maleate involves several steps, starting with the preparation of the core structure, followed by the introduction of various functional groups. The industrial production methods typically involve the use of organic solvents and reagents under controlled conditions to ensure high yield and purity. One method involves dissolving the compound in dimethyl sulfoxide, followed by the addition of polyethylene glycol and Tween 80, and finally mixing with deionized water .
Chemical Reactions Analysis
Milciclib Maleate undergoes various chemical reactions, including:
Oxidation: This reaction involves the addition of oxygen or the removal of hydrogen. Common reagents include hydrogen peroxide and potassium permanganate.
Reduction: This reaction involves the addition of hydrogen or the removal of oxygen. Common reagents include lithium aluminum hydride and sodium borohydride.
Substitution: This reaction involves the replacement of one atom or group of atoms with another. Common reagents include halogens and nucleophiles.
The major products formed from these reactions depend on the specific conditions and reagents used .
Comparison with Similar Compounds
Milciclib Maleate is unique in its ability to inhibit multiple kinases simultaneously, making it a potent antineoplastic agent. Similar compounds include:
Palbociclib: Another cyclin-dependent kinase inhibitor used in the treatment of breast cancer.
Ribociclib: A cyclin-dependent kinase inhibitor used in combination with other therapies for breast cancer.
Abemaciclib: A cyclin-dependent kinase inhibitor used for the treatment of breast cancer.
Compared to these compounds, this compound Maleate has shown broader kinase inhibition and potential efficacy in a wider range of cancers .
Properties
{ "Design of the Synthesis Pathway": "Milciclib can be synthesized via a multi-step process that involves the reaction of several starting materials to form the final product. The synthesis pathway involves the coupling of two key intermediates, which are prepared separately and then combined to form Milciclib.", "Starting Materials": [ "2-chloro-4-iodopyrimidine", "2,6-dichloro-4-(3,4-dimethoxyphenyl)pyrimidine", "sodium hydride", "N,N-dimethylformamide", "triethylamine", "palladium(II) acetate", "copper(II) sulfate pentahydrate", "sodium ascorbate", "acetic acid", "sodium hydroxide", "ethanol" ], "Reaction": [ "Step 1: Preparation of 2-chloro-4-iodopyrimidine by reacting 2-chloropyrimidine with sodium iodide in the presence of copper(II) sulfate pentahydrate and sodium ascorbate.", "Step 2: Preparation of 2,6-dichloro-4-(3,4-dimethoxyphenyl)pyrimidine by reacting 2,6-dichloropyrimidine with 3,4-dimethoxybenzaldehyde in the presence of sodium hydride and N,N-dimethylformamide.", "Step 3: Coupling of the two intermediates prepared in steps 1 and 2 by reacting them in the presence of palladium(II) acetate, triethylamine, and acetic acid in N,N-dimethylformamide.", "Step 4: Purification of the crude product obtained in step 3 by column chromatography using a mixture of ethanol and ethyl acetate as the eluent to obtain Milciclib in pure form." ] } | |
802539-81-7 | |
Molecular Formula |
C29H36N8O5 |
Molecular Weight |
576.6 g/mol |
IUPAC Name |
(Z)-but-2-enedioic acid;N,1,4,4-tetramethyl-8-[4-(4-methylpiperazin-1-yl)anilino]-5H-pyrazolo[4,3-h]quinazoline-3-carboxamide |
InChI |
InChI=1S/C25H32N8O.C4H4O4/c1-25(2)14-16-15-27-24(29-20(16)22-19(25)21(23(34)26-3)30-32(22)5)28-17-6-8-18(9-7-17)33-12-10-31(4)11-13-33;5-3(6)1-2-4(7)8/h6-9,15H,10-14H2,1-5H3,(H,26,34)(H,27,28,29);1-2H,(H,5,6)(H,7,8)/b;2-1- |
InChI Key |
DGVCEXQFNYYRQI-BTJKTKAUSA-N |
Isomeric SMILES |
CC1(CC2=CN=C(N=C2C3=C1C(=NN3C)C(=O)NC)NC4=CC=C(C=C4)N5CCN(CC5)C)C.C(=C\C(=O)O)\C(=O)O |
SMILES |
CC1(CC2=CN=C(N=C2C3=C1C(=NN3C)C(=O)NC)NC4=CC=C(C=C4)N5CCN(CC5)C)C |
Canonical SMILES |
CC1(CC2=CN=C(N=C2C3=C1C(=NN3C)C(=O)NC)NC4=CC=C(C=C4)N5CCN(CC5)C)C.C(=CC(=O)O)C(=O)O |
Appearance |
Solid powder |
802539-81-7 | |
Purity |
>98% (or refer to the Certificate of Analysis) |
shelf_life |
>2 years if stored properly |
solubility |
Soluble in DMSO, not in water |
storage |
Dry, dark and at 0 - 4 C for short term (days to weeks) or -20 C for long term (months to years). |
synonyms |
miliciclib N,1,4,4-tetramethyl-8-((4-(4-methylpiperazin-1-yl)phenyl)amino)-4,5-dihydro-1H-pyrazolo(4,3-h)quinazoline-3-carboxamide PHA 848125 PHA-848125 PHA-848125AC PHA848125 |
Origin of Product |
United States |
Retrosynthesis Analysis
AI-Powered Synthesis Planning: Our tool employs the Template_relevance Pistachio, Template_relevance Bkms_metabolic, Template_relevance Pistachio_ringbreaker, Template_relevance Reaxys, Template_relevance Reaxys_biocatalysis model, leveraging a vast database of chemical reactions to predict feasible synthetic routes.
One-Step Synthesis Focus: Specifically designed for one-step synthesis, it provides concise and direct routes for your target compounds, streamlining the synthesis process.
Accurate Predictions: Utilizing the extensive PISTACHIO, BKMS_METABOLIC, PISTACHIO_RINGBREAKER, REAXYS, REAXYS_BIOCATALYSIS database, our tool offers high-accuracy predictions, reflecting the latest in chemical research and data.
Strategy Settings
Precursor scoring | Relevance Heuristic |
---|---|
Min. plausibility | 0.01 |
Model | Template_relevance |
Template Set | Pistachio/Bkms_metabolic/Pistachio_ringbreaker/Reaxys/Reaxys_biocatalysis |
Top-N result to add to graph | 6 |
Feasible Synthetic Routes
Disclaimer and Information on In-Vitro Research Products
Please be aware that all articles and product information presented on BenchChem are intended solely for informational purposes. The products available for purchase on BenchChem are specifically designed for in-vitro studies, which are conducted outside of living organisms. In-vitro studies, derived from the Latin term "in glass," involve experiments performed in controlled laboratory settings using cells or tissues. It is important to note that these products are not categorized as medicines or drugs, and they have not received approval from the FDA for the prevention, treatment, or cure of any medical condition, ailment, or disease. We must emphasize that any form of bodily introduction of these products into humans or animals is strictly prohibited by law. It is essential to adhere to these guidelines to ensure compliance with legal and ethical standards in research and experimentation.