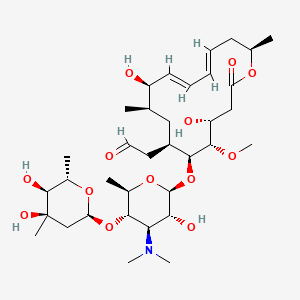
Kitasamycin
Overview
Description
Kitasamycin (also known as Leucomycin) is a 16-membered macrolide antibiotic produced by Streptomyces kitasatoensis. It comprises multiple components, including A1, A3, A4, A5, A6, A7, and A8, which contribute to its broad-spectrum activity . This compound inhibits bacterial protein synthesis by binding to the 50S ribosomal subunit, making it effective against Gram-positive bacteria, Mycoplasmas, and some Gram-negative pathogens. Notably, it retains activity against strains resistant to penicillin, erythromycin, and chloramphenicol, without inducing cross-resistance . It is widely used in veterinary medicine for treating respiratory and gastrointestinal infections in livestock and as a growth promoter in poultry and swine .
Preparation Methods
Synthetic Routes and Reaction Conditions: Kitasamycin is primarily produced through fermentation. The fermentation medium typically includes glucose, soybean cake powder, starch, ammonium chloride, monopotassium phosphate, magnesium sulfate, zinc sulfate, calcium carbonate, and soybean oil . The fermentation process involves inoculating a seed culture solution into the fermentation medium and maintaining specific conditions to optimize the production of this compound .
Industrial Production Methods: For industrial production, the preparation of this compound involves several steps. Initially, the original strain is subjected to plate streaking to prepare a single colony. This colony is then transferred to an inclined plane for further culturing. Spores are scraped from the inclined plane and used to prepare a freeze-dried strain tube. The spores are then transferred to a seed culture medium for shake culture, followed by transfer to a seed tank . This method ensures consistency in the quality of the produced this compound and is suitable for large-scale production .
Chemical Reactions Analysis
Types of Reactions: Kitasamycin undergoes various chemical reactions, including oxidation, reduction, and substitution. These reactions are essential for modifying the compound to enhance its efficacy or reduce its toxicity.
Common Reagents and Conditions:
Oxidation: Common oxidizing agents such as hydrogen peroxide or potassium permanganate can be used to oxidize this compound.
Reduction: Reducing agents like sodium borohydride or lithium aluminum hydride are employed to reduce this compound.
Substitution: Substitution reactions often involve nucleophiles such as amines or thiols under basic conditions.
Major Products: The major products formed from these reactions depend on the specific reagents and conditions used. For example, oxidation may yield hydroxylated derivatives, while reduction can produce deoxygenated forms of this compound.
Scientific Research Applications
Antimicrobial Applications
Clinical Use in Human Medicine
Kitasamycin exhibits significant efficacy against several pathogens, particularly Gram-positive cocci. It has been shown to be effective against clinical isolates of Staphylococcus aureus, Streptococcus pyogenes, and Diplococcus pneumoniae . In clinical settings, this compound has been used to treat respiratory infections and skin infections, demonstrating better outcomes than some alternative antibiotics .
Veterinary Applications
In veterinary medicine, this compound is employed to manage infections in livestock. Studies have indicated its effectiveness against various bacterial strains responsible for respiratory diseases in pigs and poultry. The minimum inhibitory concentrations (MICs) for different pathogens have been documented, showing its potency in treating infections like Actinobacillus pleuropneumoniae .
Pathogen | MIC (µg/ml) | Application |
---|---|---|
Staphylococcus aureus | 0.5 - 2 | Respiratory infections |
Streptococcus pyogenes | 0.25 - 1 | Skin infections |
Actinobacillus pleuropneumoniae | 16 - 32 | Veterinary use |
Agricultural Applications
This compound is also utilized in agriculture as a growth promoter and for disease prevention in livestock. Its ability to modulate gut microbiota has been studied, revealing that it can enhance nutrient absorption while reducing the incidence of diarrhea in weaned piglets . However, concerns regarding antibiotic resistance necessitate careful monitoring of its use.
Fibrosis Management
Recent research has indicated that this compound may have potential applications in managing fibrosis. In vitro studies demonstrated that this compound could inhibit the transformation of fibroblasts into myofibroblasts, which are key players in fibrotic processes . This suggests a novel therapeutic avenue for conditions characterized by excessive fibrosis, such as post-surgical complications.
Combination Therapy Studies
This compound's interactions with other antibiotics have been extensively studied to optimize treatment regimens. For example, its combination with enrofloxacin showed antagonistic effects against certain bacterial serotypes . Understanding these interactions can help clinicians tailor antibiotic therapy more effectively.
Combination Partner | Effect Observed | Pathogen |
---|---|---|
Enrofloxacin | Antagonistic | Actinobacillus pleuropneumoniae |
Oxolinic Acid | Synergistic-Indifferent | Various serotypes |
Case Studies
- Respiratory Infections : A clinical trial involving 81 patients treated with this compound for respiratory infections reported high rates of recovery compared to standard treatments . This underscores its efficacy in real-world settings.
- Fibrosis Prevention : In an experimental model assessing the effects of this compound on fibroblast activity, significant reductions in fibrotic markers were observed, indicating its potential role in preventing fibrosis post-surgery .
Mechanism of Action
Kitasamycin exerts its effects by inhibiting bacterial protein synthesis. It binds reversibly to the 50S subunit of the bacterial ribosome, preventing the translocation of peptidyl transfer RNA (tRNA) and thus inhibiting protein synthesis . This action is primarily bacteriostatic but can be bactericidal at higher concentrations . The molecular targets include the 23S ribosomal RNA within the 50S subunit .
Comparison with Similar Compounds
Comparative Analysis with Similar Macrolides
Structural and Functional Comparisons
Kitasamycin belongs to the 16-membered macrolide subclass, distinguishing it from 14-membered macrolides like erythromycin and 15-membered azithromycin. Key structural analogs include:
- Tylosin : A 16-membered macrolide used in veterinary medicine, effective against Mycoplasma. Both tylosin and this compound are used as growth promoters, but tylosin has a shorter withdrawal period in poultry .
- Josamycin and Midecamycin : 16-membered macrolides with similar spectra but varying pharmacokinetic profiles. Josamycin propionate exhibits improved oral bioavailability compared to this compound .
- Erythromycin : A 14-membered macrolide with broader human clinical use. This compound shows comparable efficacy but is less prone to inducing macrolide-lincosamide-streptogramin B (MLSB) resistance .
Antimicrobial Activity
Minimum Inhibitory Concentration (MIC) Profiles
This compound exhibits higher MICs than fluoroquinolones (e.g., enrofloxacin) against A. pleuropneumoniae serotypes 1, 3, 5, and 7, indicating lower potency in monotherapy . However, its subinhibitory concentrations uniquely inhibit quorum sensing (QS) in Chromobacterium violaceum, reducing virulence factors like biofilm formation and violacein production .
Pharmacokinetics and Residues
- This compound vs. Acetylthis compound : Acetylthis compound, a semi-synthetic derivative, shows higher plasma concentrations in swine (up to 12-fold) and prolonged tissue retention, enhancing therapeutic efficacy .
- Tissue Residues : this compound residues in broilers persist in bile and cecum for 3 days post-administration, necessitating a 3-day withdrawal period before slaughter . Comparable macrolides like tylosin have shorter withdrawal periods (1–2 days) .
Combination Therapy Interactions
This compound’s interactions with other antibiotics are pathogen-dependent:
- Antagonism with Enrofloxacin : Observed against all A. pleuropneumoniae serotypes due to conflicting mechanisms (protein synthesis vs. DNA gyrase inhibition) .
- Indifference with Norfloxacin: No enhanced or reduced efficacy across serotypes .
In contrast, erythromycin and fluoroquinolones often exhibit additive effects in treating respiratory infections, highlighting structural class differences in interaction profiles .
Unique Non-Antibiotic Effects
- Growth Promotion: Subtherapeutic doses enhance fat accumulation in swine muscle, improving meat quality metrics.
Market and Regulatory Landscape
The global this compound market (CAGR 5.1%) is driven by veterinary use, outpacing erythromycin in livestock applications. Key trends include:
Q & A
Basic Research Questions
Q. How to experimentally determine the optimal concentration of precursors (e.g., ethyl acetate) for enhancing kitasamycin biosynthesis in Streptomyces kitasatoensis?
- Methodology :
- Use shake-flask fermentation with varying precursor concentrations (e.g., 0.32%–0.96% ethyl acetate) and measure this compound titers via bioactivity assays (e.g., agar diffusion).
- Monitor cell growth (OD600) and precursor decomposition kinetics to avoid growth inhibition .
- Validate findings in scaled-up fermentors (e.g., 15-L) to assess industrial feasibility .
Q. What analytical methods are recommended for quantifying this compound components (e.g., A4 vs. A5) and ensuring purity in research samples?
- Methodology :
- Employ high-performance liquid chromatography (HPLC) with UV detection at 231 nm, calibrated against leucomycin A5 reference standards .
- Use ion chromatography for quantifying organic acids (e.g., tartaric acid) in formulations, correlating pH and clarity to validate salt-forming rates .
Q. Why does ethyl acetate outperform sodium acetate as a precursor in this compound biosynthesis?
- Methodology :
- Compare intracellular acetyl-CoA levels and enzyme activities (acyl-CoA synthetase, acyl-kinase) between precursor treatments via enzymatic assays .
- Analyze short-chain fatty acid accumulation (e.g., butyrate) using gas chromatography to link precursor metabolism to A5/A4 ratios .
Advanced Research Questions
Q. How does ethyl acetate regulate acetyltransferase activity and suppress A5-to-A4 conversion during this compound biosynthesis?
- Methodology :
- Conduct time-course enzyme assays (AS, AK, APT) under ethyl acetate supplementation to identify peak activity phases .
- Use RNA-seq to profile acetyltransferase gene expression (e.g., act genes) and validate via CRISPR-Cas9 knockouts .
- Quantify intracellular butyrate levels via LC-MS to confirm its role in inhibiting C-3 acetylation .
Q. What experimental strategies resolve contradictions in this compound titers at high precursor concentrations (e.g., >0.64% ethyl acetate)?
- Methodology :
- Perform metabolic flux analysis (13C-labeling) to identify bottlenecks in lactonic ring biosynthesis or acetyl-CoA utilization .
- Test combinatorial precursor supplementation (e.g., butyrate + ethyl acetate) to bypass metabolic limitations .
Q. How to elucidate the unclear biosynthetic pathway of A5-to-A4 conversion in S. kitasatoensis?
- Methodology :
- Isolate intermediates via preparative HPLC and characterize structures via NMR and HR-MS .
- Use heterologous expression of putative acetyltransferase genes in E. coli to reconstitute A4 synthesis in vitro .
Q. Data Contradiction Analysis
Q. Why does sodium acetate inconsistently enhance this compound titers despite being a known precursor?
- Resolution :
- Sodium acetate disrupts cell growth at >0.15% due to pH instability, whereas ethyl acetate slowly releases acetic acid without growth inhibition .
- Sodium acetate fails to induce acyl-kinase (AK) activity, limiting acetyl-phosphate production for lactonic ring formation .
Q. Key Research Gaps
Properties
IUPAC Name |
2-[(11E,13Z)-6-[5-(4,5-dihydroxy-4,6-dimethyloxan-2-yl)oxy-4-(dimethylamino)-3-hydroxy-6-methyloxan-2-yl]oxy-4,10-dihydroxy-5-methoxy-9,16-dimethyl-2-oxo-1-oxacyclohexadeca-11,13-dien-7-yl]acetaldehyde | |
---|---|---|
Details | Computed by Lexichem TK 2.7.0 (PubChem release 2021.10.14) | |
Source | PubChem | |
URL | https://pubchem.ncbi.nlm.nih.gov | |
Description | Data deposited in or computed by PubChem | |
InChI |
InChI=1S/C35H59NO13/c1-19-16-23(14-15-37)31(32(44-8)25(39)17-26(40)45-20(2)12-10-9-11-13-24(19)38)49-34-29(41)28(36(6)7)30(21(3)47-34)48-27-18-35(5,43)33(42)22(4)46-27/h9-11,13,15,19-25,27-34,38-39,41-43H,12,14,16-18H2,1-8H3/b10-9-,13-11+ | |
Details | Computed by InChI 1.0.6 (PubChem release 2021.10.14) | |
Source | PubChem | |
URL | https://pubchem.ncbi.nlm.nih.gov | |
Description | Data deposited in or computed by PubChem | |
InChI Key |
XYJOGTQLTFNMQG-AZQRDTPRSA-N | |
Details | Computed by InChI 1.0.6 (PubChem release 2021.10.14) | |
Source | PubChem | |
URL | https://pubchem.ncbi.nlm.nih.gov | |
Description | Data deposited in or computed by PubChem | |
Canonical SMILES |
CC1CC=CC=CC(C(CC(C(C(C(CC(=O)O1)O)OC)OC2C(C(C(C(O2)C)OC3CC(C(C(O3)C)O)(C)O)N(C)C)O)CC=O)C)O | |
Details | Computed by OEChem 2.3.0 (PubChem release 2021.10.14) | |
Source | PubChem | |
URL | https://pubchem.ncbi.nlm.nih.gov | |
Description | Data deposited in or computed by PubChem | |
Isomeric SMILES |
CC1C/C=C\C=C\C(C(CC(C(C(C(CC(=O)O1)O)OC)OC2C(C(C(C(O2)C)OC3CC(C(C(O3)C)O)(C)O)N(C)C)O)CC=O)C)O | |
Details | Computed by OEChem 2.3.0 (PubChem release 2021.10.14) | |
Source | PubChem | |
URL | https://pubchem.ncbi.nlm.nih.gov | |
Description | Data deposited in or computed by PubChem | |
Molecular Formula |
C35H59NO13 | |
Details | Computed by PubChem 2.2 (PubChem release 2021.10.14) | |
Source | PubChem | |
URL | https://pubchem.ncbi.nlm.nih.gov | |
Description | Data deposited in or computed by PubChem | |
Molecular Weight |
701.8 g/mol | |
Details | Computed by PubChem 2.2 (PubChem release 2021.10.14) | |
Source | PubChem | |
URL | https://pubchem.ncbi.nlm.nih.gov | |
Description | Data deposited in or computed by PubChem | |
Retrosynthesis Analysis
AI-Powered Synthesis Planning: Our tool employs the Template_relevance Pistachio, Template_relevance Bkms_metabolic, Template_relevance Pistachio_ringbreaker, Template_relevance Reaxys, Template_relevance Reaxys_biocatalysis model, leveraging a vast database of chemical reactions to predict feasible synthetic routes.
One-Step Synthesis Focus: Specifically designed for one-step synthesis, it provides concise and direct routes for your target compounds, streamlining the synthesis process.
Accurate Predictions: Utilizing the extensive PISTACHIO, BKMS_METABOLIC, PISTACHIO_RINGBREAKER, REAXYS, REAXYS_BIOCATALYSIS database, our tool offers high-accuracy predictions, reflecting the latest in chemical research and data.
Strategy Settings
Precursor scoring | Relevance Heuristic |
---|---|
Min. plausibility | 0.01 |
Model | Template_relevance |
Template Set | Pistachio/Bkms_metabolic/Pistachio_ringbreaker/Reaxys/Reaxys_biocatalysis |
Top-N result to add to graph | 6 |
Feasible Synthetic Routes
Disclaimer and Information on In-Vitro Research Products
Please be aware that all articles and product information presented on BenchChem are intended solely for informational purposes. The products available for purchase on BenchChem are specifically designed for in-vitro studies, which are conducted outside of living organisms. In-vitro studies, derived from the Latin term "in glass," involve experiments performed in controlled laboratory settings using cells or tissues. It is important to note that these products are not categorized as medicines or drugs, and they have not received approval from the FDA for the prevention, treatment, or cure of any medical condition, ailment, or disease. We must emphasize that any form of bodily introduction of these products into humans or animals is strictly prohibited by law. It is essential to adhere to these guidelines to ensure compliance with legal and ethical standards in research and experimentation.