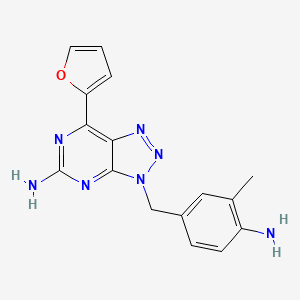
Vipadenant
Overview
Description
Vipadenant is a selective antagonist of the adenosine A2a receptor. It was initially developed by Vernalis as an oral drug for the treatment of Parkinson’s disease. The compound has shown potential in disrupting immunosuppressive mechanisms in tumor protection, making it a promising candidate for combination therapies in cancer treatment .
Mechanism of Action
Target of Action
Vipadenant is a small molecule that primarily targets the adenosine A2a receptor (A2aR) . The A2a receptor plays a critical role in the immune checkpoint pathways, which have been emphasized in many studies as novel anticancer strategies .
Mode of Action
This compound acts as an antagonist to the A2a receptor . An antagonist is a substance that interferes with or inhibits the physiological action of another. In this case, this compound inhibits the action of the A2a receptor, thereby modulating its function .
Biochemical Pathways
It is known that the a2a receptor plays a critical role in activating the negative immunity feedback loop in the tumor microenvironment . Therefore, by acting as an antagonist to the A2a receptor, this compound could potentially disrupt this feedback loop and enhance the body’s immune response against tumors .
Pharmacokinetics
The pharmacokinetics of this compound have been studied in rats using a liquid chromatography–quadrupole-time-of-flight–mass spectrometric (LC-QTOF-MS) assay . The study showed that this compound has a bioavailability of 30.4 ± 8.9% in rats, with a low to moderate drug clearance .
Result of Action
As an antagonist of the a2a receptor, this compound is expected to modulate the function of this receptor and potentially enhance the body’s immune response against tumors .
Biochemical Analysis
Biochemical Properties
Vipadenant functions as an antagonist of the adenosine A2a receptor, a G protein-coupled receptor that mediates various physiological processes. By binding to this receptor, this compound inhibits the action of adenosine, a nucleoside that modulates neurotransmission, inflammation, and immune responses . The interaction between this compound and the A2a receptor prevents the activation of downstream signaling pathways that would otherwise lead to immunosuppression in the tumor microenvironment . Additionally, this compound has been shown to interact with other biomolecules, including enzymes involved in its metabolism, such as cytochrome P450 .
Cellular Effects
This compound exerts significant effects on various cell types, particularly immune cells. By blocking the A2a receptor, this compound enhances the activity of cytotoxic T cells and natural killer cells, promoting an anti-tumor immune response . This compound also influences cell signaling pathways, such as the cyclic AMP (cAMP) pathway, which is involved in regulating immune cell function . Furthermore, this compound affects gene expression by modulating the transcription of genes associated with immune activation and inflammation .
Molecular Mechanism
At the molecular level, this compound binds to the adenosine A2a receptor, preventing adenosine from activating this receptor . This inhibition blocks the downstream signaling cascade, which includes the activation of adenylate cyclase and the subsequent increase in cAMP levels . By reducing cAMP levels, this compound diminishes the immunosuppressive effects of adenosine, thereby enhancing the immune response against tumors . Additionally, this compound may influence the expression of genes involved in immune regulation through its effects on transcription factors .
Temporal Effects in Laboratory Settings
In laboratory settings, the effects of this compound have been observed to change over time. Studies have shown that this compound is relatively stable, with a moderate rate of degradation . Long-term exposure to this compound in vitro and in vivo has demonstrated sustained enhancement of immune cell activity and anti-tumor effects . The stability and efficacy of this compound may vary depending on the experimental conditions and the duration of treatment .
Dosage Effects in Animal Models
The effects of this compound vary with different dosages in animal models. At lower doses, this compound effectively enhances immune cell activity without causing significant toxicity . At higher doses, this compound may induce adverse effects, such as hepatotoxicity and gastrointestinal disturbances . The optimal dosage of this compound for therapeutic use requires careful consideration to balance efficacy and safety .
Metabolic Pathways
This compound is metabolized primarily by the liver, involving enzymes such as cytochrome P450 . The metabolic pathways of this compound include oxidation, reduction, and conjugation reactions, leading to the formation of various metabolites . These metabolites may have different pharmacological activities and contribute to the overall effects of this compound . Understanding the metabolic pathways of this compound is crucial for optimizing its therapeutic use and minimizing potential side effects .
Transport and Distribution
This compound is transported and distributed within cells and tissues through various mechanisms. It can cross cell membranes and accumulate in specific tissues, such as the liver and spleen . Transporters and binding proteins, such as albumin, may facilitate the distribution of this compound within the body . The localization and accumulation of this compound in target tissues are essential for its therapeutic efficacy .
Subcellular Localization
The subcellular localization of this compound is primarily within the cytoplasm, where it interacts with the adenosine A2a receptor . This compound may also localize to other cellular compartments, such as the endoplasmic reticulum and mitochondria, depending on its interactions with specific biomolecules . The subcellular distribution of this compound can influence its activity and function, affecting its overall therapeutic potential .
Preparation Methods
Synthetic Routes and Reaction Conditions
The synthesis of vipadenant involves multiple steps, including the formation of a triazolopyrimidine core. The key steps typically involve:
Formation of the triazole ring: This is achieved through a cyclization reaction involving appropriate precursors.
Substitution reactions: Various substituents are introduced to the triazole ring to form the final compound.
Industrial Production Methods
Industrial production of this compound involves scaling up the synthetic routes mentioned above. The process includes optimization of reaction conditions to ensure high yield and purity. Common techniques used include:
Liquid chromatography: For purification of intermediates and the final product.
Mass spectrometry: For monitoring the reaction progress and ensuring the quality of the final product.
Chemical Reactions Analysis
Types of Reactions
Vipadenant undergoes several types of chemical reactions, including:
Oxidation: This reaction can modify the functional groups on the molecule.
Reduction: Used to reduce specific functional groups.
Substitution: Introduction of various substituents to the triazole ring.
Common Reagents and Conditions
Oxidizing agents: Such as hydrogen peroxide or potassium permanganate.
Reducing agents: Such as sodium borohydride.
Substituents: Various halides and other electrophiles.
Major Products
The major products formed from these reactions include various derivatives of this compound, which can be used for further research and development .
Scientific Research Applications
Vipadenant has a wide range of scientific research applications:
Chemistry: Used as a model compound for studying adenosine receptor antagonists.
Biology: Investigated for its effects on cellular signaling pathways.
Medicine: Explored as a potential treatment for Parkinson’s disease and as an adjunct in cancer immunotherapy.
Industry: Used in the development of new pharmaceuticals and therapeutic agents.
Comparison with Similar Compounds
Similar Compounds
Istradefylline: Another adenosine A2a receptor antagonist used in the treatment of Parkinson’s disease.
Preladenant: A selective adenosine A2a receptor antagonist investigated for its potential in treating Parkinson’s disease.
Tozadenant: Another compound in the same class, with similar therapeutic applications.
Uniqueness
Vipadenant is unique due to its high selectivity for the adenosine A2a receptor and its potential use in combination therapies for cancer treatment. Unlike some other compounds, this compound has shown promising results in preclinical and clinical studies for both neurological and oncological applications .
Properties
IUPAC Name |
3-[(4-amino-3-methylphenyl)methyl]-7-(furan-2-yl)triazolo[4,5-d]pyrimidin-5-amine | |
---|---|---|
Source | PubChem | |
URL | https://pubchem.ncbi.nlm.nih.gov | |
Description | Data deposited in or computed by PubChem | |
InChI |
InChI=1S/C16H15N7O/c1-9-7-10(4-5-11(9)17)8-23-15-14(21-22-23)13(19-16(18)20-15)12-3-2-6-24-12/h2-7H,8,17H2,1H3,(H2,18,19,20) | |
Source | PubChem | |
URL | https://pubchem.ncbi.nlm.nih.gov | |
Description | Data deposited in or computed by PubChem | |
InChI Key |
HQSBCDPYXDGTCL-UHFFFAOYSA-N | |
Source | PubChem | |
URL | https://pubchem.ncbi.nlm.nih.gov | |
Description | Data deposited in or computed by PubChem | |
Canonical SMILES |
CC1=C(C=CC(=C1)CN2C3=NC(=NC(=C3N=N2)C4=CC=CO4)N)N | |
Source | PubChem | |
URL | https://pubchem.ncbi.nlm.nih.gov | |
Description | Data deposited in or computed by PubChem | |
Molecular Formula |
C16H15N7O | |
Source | PubChem | |
URL | https://pubchem.ncbi.nlm.nih.gov | |
Description | Data deposited in or computed by PubChem | |
DSSTOX Substance ID |
DTXSID90196103 | |
Record name | Vipadenant | |
Source | EPA DSSTox | |
URL | https://comptox.epa.gov/dashboard/DTXSID90196103 | |
Description | DSSTox provides a high quality public chemistry resource for supporting improved predictive toxicology. | |
Molecular Weight |
321.34 g/mol | |
Source | PubChem | |
URL | https://pubchem.ncbi.nlm.nih.gov | |
Description | Data deposited in or computed by PubChem | |
CAS No. |
442908-10-3 | |
Record name | Vipadenant [USAN:INN] | |
Source | ChemIDplus | |
URL | https://pubchem.ncbi.nlm.nih.gov/substance/?source=chemidplus&sourceid=0442908103 | |
Description | ChemIDplus is a free, web search system that provides access to the structure and nomenclature authority files used for the identification of chemical substances cited in National Library of Medicine (NLM) databases, including the TOXNET system. | |
Record name | Vipadenant | |
Source | DrugBank | |
URL | https://www.drugbank.ca/drugs/DB06625 | |
Description | The DrugBank database is a unique bioinformatics and cheminformatics resource that combines detailed drug (i.e. chemical, pharmacological and pharmaceutical) data with comprehensive drug target (i.e. sequence, structure, and pathway) information. | |
Explanation | Creative Common's Attribution-NonCommercial 4.0 International License (http://creativecommons.org/licenses/by-nc/4.0/legalcode) | |
Record name | Vipadenant | |
Source | EPA DSSTox | |
URL | https://comptox.epa.gov/dashboard/DTXSID90196103 | |
Description | DSSTox provides a high quality public chemistry resource for supporting improved predictive toxicology. | |
Record name | VIPADENANT | |
Source | FDA Global Substance Registration System (GSRS) | |
URL | https://gsrs.ncats.nih.gov/ginas/app/beta/substances/LDR3USH1NJ | |
Description | The FDA Global Substance Registration System (GSRS) enables the efficient and accurate exchange of information on what substances are in regulated products. Instead of relying on names, which vary across regulatory domains, countries, and regions, the GSRS knowledge base makes it possible for substances to be defined by standardized, scientific descriptions. | |
Explanation | Unless otherwise noted, the contents of the FDA website (www.fda.gov), both text and graphics, are not copyrighted. They are in the public domain and may be republished, reprinted and otherwise used freely by anyone without the need to obtain permission from FDA. Credit to the U.S. Food and Drug Administration as the source is appreciated but not required. | |
Retrosynthesis Analysis
AI-Powered Synthesis Planning: Our tool employs the Template_relevance Pistachio, Template_relevance Bkms_metabolic, Template_relevance Pistachio_ringbreaker, Template_relevance Reaxys, Template_relevance Reaxys_biocatalysis model, leveraging a vast database of chemical reactions to predict feasible synthetic routes.
One-Step Synthesis Focus: Specifically designed for one-step synthesis, it provides concise and direct routes for your target compounds, streamlining the synthesis process.
Accurate Predictions: Utilizing the extensive PISTACHIO, BKMS_METABOLIC, PISTACHIO_RINGBREAKER, REAXYS, REAXYS_BIOCATALYSIS database, our tool offers high-accuracy predictions, reflecting the latest in chemical research and data.
Strategy Settings
Precursor scoring | Relevance Heuristic |
---|---|
Min. plausibility | 0.01 |
Model | Template_relevance |
Template Set | Pistachio/Bkms_metabolic/Pistachio_ringbreaker/Reaxys/Reaxys_biocatalysis |
Top-N result to add to graph | 6 |
Feasible Synthetic Routes
Disclaimer and Information on In-Vitro Research Products
Please be aware that all articles and product information presented on BenchChem are intended solely for informational purposes. The products available for purchase on BenchChem are specifically designed for in-vitro studies, which are conducted outside of living organisms. In-vitro studies, derived from the Latin term "in glass," involve experiments performed in controlled laboratory settings using cells or tissues. It is important to note that these products are not categorized as medicines or drugs, and they have not received approval from the FDA for the prevention, treatment, or cure of any medical condition, ailment, or disease. We must emphasize that any form of bodily introduction of these products into humans or animals is strictly prohibited by law. It is essential to adhere to these guidelines to ensure compliance with legal and ethical standards in research and experimentation.