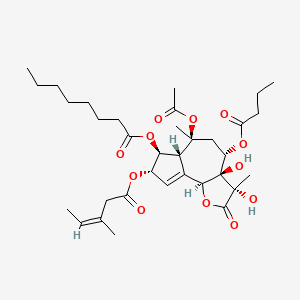
Thapsigargin
Overview
Description
Scientific Research Applications
Thapsigargin has a wide range of scientific research applications:
Chemistry: It is used as a tool to study the importance of SERCA in intracellular calcium homeostasis .
Biology: this compound is employed in experiments to examine the impacts of increasing cytosolic calcium concentrations and endoplasmic reticulum calcium depletion .
Medicine: this compound has shown potential as an anticancer agent due to its ability to induce apoptosis by inhibiting the SERCA pump . It has also been investigated for its use against coronavirus infections .
Industry: The compound is used in the development of prodrugs for targeted cancer therapy .
Mechanism of Action
Target of Action
Thapsigargin primarily targets the sarco/endoplasmic reticulum Ca2+ ATPase (SERCA) . SERCA is a pump protein that is essential for cellular viability, and it plays a crucial role in intracellular calcium homeostasis . This compound also has a minor effect on the secretory pathway Ca2+/Mn2+ ATPase (SPCA) pump .
Mode of Action
This compound acts as a non-competitive inhibitor of SERCA . By inhibiting SERCA, this compound blocks the ability of the cell to pump calcium into the sarcoplasmic and endoplasmic reticula . This leads to an increase in cytosolic (intracellular) calcium concentration . The increase in cytosolic calcium concentration can activate plasma membrane calcium channels, allowing an influx of calcium into the cytosol .
Biochemical Pathways
The inhibition of SERCA by this compound leads to the depletion of calcium stores in the endoplasmic reticulum (ER), which triggers the unfolded protein response (UPR) . The UPR is a cellular stress response related to the ER. It is activated in response to an accumulation of unfolded or misfolded proteins in the lumen of the ER . This compound-induced ER stress can lead to cell death .
Pharmacokinetics
The pharmacokinetics of this compound are still under investigation. A prodrug of this compound, known as mipsagargin (g-202), has been developed for cancer treatment . Mipsagargin is designed to target the blood vessels of cancer cells, leading to tumor necrosis . The prodrug is activated by prostate-specific membrane antigen (PSMA)-mediated cleavage of an inert masking peptide .
Result of Action
The action of this compound results in a variety of molecular and cellular effects. It can lead to cell death through apoptosis . This compound treatment and the resulting ER calcium depletion can inhibit autophagy independent of the UPR . Non-resolved ER stress can cumulatively lead to cell death .
Action Environment
The action, efficacy, and stability of this compound can be influenced by various environmental factors. For instance, the lipophilic properties of the drug allow it to enter into the cellular membranes where it can interact with SERCA . Furthermore, the plant Thapsia garganica, from which this compound is derived, has been used for centuries in traditional medicine in the Mediterranean region, indicating that the compound’s action may be influenced by the specific environmental conditions of this region .
Advantages and Limitations for Lab Experiments
One advantage of using thapsigargin in experiments is its ability to induce this compound stress, allowing for the study of this important biological process. However, it is important to note that this compound is a potent compound and care should be taken to use appropriate doses and controls to avoid artifacts in experimental results.
Future Directions
For thapsigargin research include optimization of synthesis methods, identification of specific targets for this compound-mediated cell death in disease models, and exploration of this compound derivatives or analogs with improved pharmacological properties.
In conclusion, this compound is a valuable tool for understanding the this compound stress response, calcium signaling, and cell death. Its potential applications in the treatment of diseases related to these processes make it an important area of investigation, and continued research will likely lead to new therapeutic approaches for a range of diseases.
Biochemical Analysis
Biochemical Properties
Thapsigargin is a non-competitive inhibitor of the sarco/endoplasmic reticulum Ca2+ ATPase (SERCA) . It raises cytosolic (intracellular) calcium concentration by blocking the ability of the cell to pump calcium into the sarcoplasmic and endoplasmic reticula . This increase in cytoplasmic free [Ca2+] occurs in the absence of detectable production of inositol phosphates .
Cellular Effects
This compound has a profound impact on various types of cells and cellular processes. It influences cell function by altering cell signaling pathways, gene expression, and cellular metabolism . This compound treatment and the resulting ER calcium depletion inhibits autophagy independent of the unfolded protein response . It also induces a hormone-like elevation of cytosolic calcium .
Molecular Mechanism
This compound exerts its effects at the molecular level through several mechanisms. It inhibits the SERCA pump, leading to an increase in cytosolic calcium concentration . This store-depletion can secondarily activate plasma membrane calcium channels, allowing an influx of calcium into the cytosol . Depletion of ER calcium stores leads to ER stress and activation of the unfolded protein response .
Temporal Effects in Laboratory Settings
In laboratory settings, this compound-induced cell stress is detected an order of magnitude prior to any changes in cellular proliferation . Stress levels peak after 6 to 7 hours of treatment, but cells recover naturally from this stress after 22 hours .
Dosage Effects in Animal Models
This compound has shown antiviral activity in animal models of Influenza A Virus (IAV) infection . The multimodal antiviral actions of this compound define a new prototype of host-directed broad-spectrum antiviral drugs that efficiently suppress the replication of enveloped RNA viruses .
Metabolic Pathways
The full biosynthetic pathway of this compound is still not elucidated, but the first steps from FPP have been identified as catalyzed by the enzyme TgTPS2 . This results in the production of epikunzeaol, followed by TgCYP76AE2 that converts epikunzeaol to epihydrocostunolide .
Transport and Distribution
It is known that this compound is selective to the SERCA pump and to a minor extent the secretory pathway Ca2+/Mn2+ ATPase (SPCA) pump .
Subcellular Localization
This compound primarily localizes in the endoplasmic reticulum (ER) due to its interaction with the SERCA pump . Under ER-stress conditions, this compound mainly localizes in the Golgi . This localization is restored to ER localization by overexpression of ribophorin I .
Preparation Methods
Synthetic Routes and Reaction Conditions: Thapsigargin is primarily extracted from the roots and fruits of Thapsia garganica using methanol, followed by purification through high-performance liquid chromatography (HPLC) . The synthetic route involves several steps, including the use of farnesyl pyrophosphate as a starting material, with germacrene B synthase catalyzing the first step . The complete biosynthesis of this compound has yet to be fully elucidated .
Industrial Production Methods: Industrial production of this compound involves the extraction from Thapsia garganica, followed by purification using HPLC . The development of protocols for selective transformation of this compound has facilitated its total synthesis, making it economically feasible for large-scale production .
Chemical Reactions Analysis
Types of Reactions: Thapsigargin undergoes various chemical reactions, including oxidation, reduction, and substitution .
Common Reagents and Conditions: Common reagents used in these reactions include methanol, ethanol, and acetonitrile . The conditions often involve room temperature reactions with specific catalysts and solvents .
Major Products: The major products formed from these reactions include derivatives of this compound, such as thapsigargicin and nortrilobolide .
Comparison with Similar Compounds
Biological Activity
Thapsigargin, a sesquiterpene lactone derived from the plant Thapsia garganica, has garnered attention for its potent biological activities, particularly in cancer research and cellular signaling. This article explores the diverse biological effects of this compound, focusing on its mechanisms of action, therapeutic potential, and case studies that highlight its efficacy in various cancer types.
This compound functions primarily as an inhibitor of the sarco/endoplasmic reticulum Ca²⁺-ATPase (SERCA) pump. By inhibiting SERCA, this compound disrupts calcium homeostasis within the endoplasmic reticulum (ER), leading to increased cytosolic calcium levels and subsequent activation of stress responses. This mechanism triggers several downstream effects:
- Induction of Apoptosis : this compound has been shown to induce apoptosis in various cancer cell lines by activating pro-apoptotic pathways and inhibiting anti-apoptotic proteins. For instance, it enhances the expression of death receptors such as DR5 and activates caspases, which are crucial for apoptosis execution .
- Endoplasmic Reticulum Stress : The disruption of calcium dynamics leads to ER stress, activating the unfolded protein response (UPR). This response can either promote cell survival or lead to apoptosis depending on the extent and duration of stress .
- Reactive Oxygen Species (ROS) Generation : this compound treatment has been associated with increased ROS production, which can further exacerbate cellular damage and promote apoptosis .
Case Studies
-
This compound in Esophageal Cancer :
A study demonstrated that this compound sensitizes esophageal squamous cell carcinoma (ESCC) to TRAIL-induced apoptosis. Treatment with this compound increased levels of CHOP and DR5, leading to enhanced apoptosis through caspase activation. The combination treatment resulted in significant increases in ROS levels and decreased GSH levels, indicating a shift in redox status conducive to apoptosis . -
Adrenocortical Carcinoma :
Research indicated that this compound inhibits proliferation and induces apoptosis in human adrenocortical carcinoma (ACC) cells via the activation of Jun N-terminal kinase (JNK) signaling pathways. In vivo studies showed that this compound significantly suppressed ACC xenograft growth, suggesting its potential as a therapeutic agent . -
Rheumatoid Arthritis :
This compound has also been investigated for its effects on fibroblast-like synoviocytes in rheumatoid arthritis. It was found to impair cell proliferation and induce apoptosis through downregulation of cyclin D1 and mTOR signaling pathways .
Data Summary
The following table summarizes key findings from various studies on this compound's biological activity:
Study Focus | Cell Type | Key Findings | Mechanism of Action |
---|---|---|---|
Esophageal Cancer | ESCC Cells | Increased apoptosis via TRAIL sensitization | Upregulation of DR5, caspase activation |
Adrenocortical Carcinoma | ACC Cells | Inhibition of proliferation; induced apoptosis | Activation of JNK signaling |
Rheumatoid Arthritis | Fibroblast-like Synoviocytes | Impaired proliferation; induced apoptosis | Downregulation of cyclin D1, mTOR signaling |
Properties
IUPAC Name |
[(3S,3aR,4S,6S,6aR,7S,8S,9bS)-6-acetyloxy-4-butanoyloxy-3,3a-dihydroxy-3,6,9-trimethyl-8-[(Z)-2-methylbut-2-enoyl]oxy-2-oxo-4,5,6a,7,8,9b-hexahydroazuleno[4,5-b]furan-7-yl] octanoate | |
---|---|---|
Source | PubChem | |
URL | https://pubchem.ncbi.nlm.nih.gov | |
Description | Data deposited in or computed by PubChem | |
InChI |
InChI=1S/C34H50O12/c1-9-12-13-14-15-17-24(37)43-28-26-25(20(5)27(28)44-30(38)19(4)11-3)29-34(41,33(8,40)31(39)45-29)22(42-23(36)16-10-2)18-32(26,7)46-21(6)35/h11,22,26-29,40-41H,9-10,12-18H2,1-8H3/b19-11-/t22-,26+,27-,28-,29-,32-,33+,34+/m0/s1 | |
Source | PubChem | |
URL | https://pubchem.ncbi.nlm.nih.gov | |
Description | Data deposited in or computed by PubChem | |
InChI Key |
IXFPJGBNCFXKPI-FSIHEZPISA-N | |
Source | PubChem | |
URL | https://pubchem.ncbi.nlm.nih.gov | |
Description | Data deposited in or computed by PubChem | |
Canonical SMILES |
CCCCCCCC(=O)OC1C2C(=C(C1OC(=O)C(=CC)C)C)C3C(C(CC2(C)OC(=O)C)OC(=O)CCC)(C(C(=O)O3)(C)O)O | |
Source | PubChem | |
URL | https://pubchem.ncbi.nlm.nih.gov | |
Description | Data deposited in or computed by PubChem | |
Isomeric SMILES |
CCCCCCCC(=O)O[C@H]1[C@H]2C(=C([C@@H]1OC(=O)/C(=C\C)/C)C)[C@H]3[C@]([C@H](C[C@]2(C)OC(=O)C)OC(=O)CCC)([C@](C(=O)O3)(C)O)O | |
Source | PubChem | |
URL | https://pubchem.ncbi.nlm.nih.gov | |
Description | Data deposited in or computed by PubChem | |
Molecular Formula |
C34H50O12 | |
Source | PubChem | |
URL | https://pubchem.ncbi.nlm.nih.gov | |
Description | Data deposited in or computed by PubChem | |
Molecular Weight |
650.8 g/mol | |
Source | PubChem | |
URL | https://pubchem.ncbi.nlm.nih.gov | |
Description | Data deposited in or computed by PubChem | |
CAS No. |
67526-95-8 | |
Record name | (-)-Thapsigargin | |
Source | CAS Common Chemistry | |
URL | https://commonchemistry.cas.org/detail?cas_rn=67526-95-8 | |
Description | CAS Common Chemistry is an open community resource for accessing chemical information. Nearly 500,000 chemical substances from CAS REGISTRY cover areas of community interest, including common and frequently regulated chemicals, and those relevant to high school and undergraduate chemistry classes. This chemical information, curated by our expert scientists, is provided in alignment with our mission as a division of the American Chemical Society. | |
Explanation | The data from CAS Common Chemistry is provided under a CC-BY-NC 4.0 license, unless otherwise stated. | |
Record name | Thapsigargin | |
Source | ChemIDplus | |
URL | https://pubchem.ncbi.nlm.nih.gov/substance/?source=chemidplus&sourceid=0067526958 | |
Description | ChemIDplus is a free, web search system that provides access to the structure and nomenclature authority files used for the identification of chemical substances cited in National Library of Medicine (NLM) databases, including the TOXNET system. | |
Record name | Octanoic acid, (3S,3aR,4S,6S,6aR,7S,8S,9bS)-6-(acetyloxy)-2,3,3a,4,5,6,6a,7,8,9b-decahydro-3,3a-dihydroxy-3,6,9-trimethyl-8-[[(2Z)-2-methyl-1-oxo-2-buten-1-yl]oxy]-2-oxo-4-(1-oxobutoxy)azuleno[4,5-b]furan-7-yl ester | |
Source | European Chemicals Agency (ECHA) | |
URL | https://echa.europa.eu/substance-information/-/substanceinfo/100.116.539 | |
Description | The European Chemicals Agency (ECHA) is an agency of the European Union which is the driving force among regulatory authorities in implementing the EU's groundbreaking chemicals legislation for the benefit of human health and the environment as well as for innovation and competitiveness. | |
Explanation | Use of the information, documents and data from the ECHA website is subject to the terms and conditions of this Legal Notice, and subject to other binding limitations provided for under applicable law, the information, documents and data made available on the ECHA website may be reproduced, distributed and/or used, totally or in part, for non-commercial purposes provided that ECHA is acknowledged as the source: "Source: European Chemicals Agency, http://echa.europa.eu/". Such acknowledgement must be included in each copy of the material. ECHA permits and encourages organisations and individuals to create links to the ECHA website under the following cumulative conditions: Links can only be made to webpages that provide a link to the Legal Notice page. | |
Record name | THAPSIGARGIN | |
Source | FDA Global Substance Registration System (GSRS) | |
URL | https://gsrs.ncats.nih.gov/ginas/app/beta/substances/Z96BQ26RZD | |
Description | The FDA Global Substance Registration System (GSRS) enables the efficient and accurate exchange of information on what substances are in regulated products. Instead of relying on names, which vary across regulatory domains, countries, and regions, the GSRS knowledge base makes it possible for substances to be defined by standardized, scientific descriptions. | |
Explanation | Unless otherwise noted, the contents of the FDA website (www.fda.gov), both text and graphics, are not copyrighted. They are in the public domain and may be republished, reprinted and otherwise used freely by anyone without the need to obtain permission from FDA. Credit to the U.S. Food and Drug Administration as the source is appreciated but not required. | |
Retrosynthesis Analysis
AI-Powered Synthesis Planning: Our tool employs the Template_relevance Pistachio, Template_relevance Bkms_metabolic, Template_relevance Pistachio_ringbreaker, Template_relevance Reaxys, Template_relevance Reaxys_biocatalysis model, leveraging a vast database of chemical reactions to predict feasible synthetic routes.
One-Step Synthesis Focus: Specifically designed for one-step synthesis, it provides concise and direct routes for your target compounds, streamlining the synthesis process.
Accurate Predictions: Utilizing the extensive PISTACHIO, BKMS_METABOLIC, PISTACHIO_RINGBREAKER, REAXYS, REAXYS_BIOCATALYSIS database, our tool offers high-accuracy predictions, reflecting the latest in chemical research and data.
Strategy Settings
Precursor scoring | Relevance Heuristic |
---|---|
Min. plausibility | 0.01 |
Model | Template_relevance |
Template Set | Pistachio/Bkms_metabolic/Pistachio_ringbreaker/Reaxys/Reaxys_biocatalysis |
Top-N result to add to graph | 6 |
Feasible Synthetic Routes
Disclaimer and Information on In-Vitro Research Products
Please be aware that all articles and product information presented on BenchChem are intended solely for informational purposes. The products available for purchase on BenchChem are specifically designed for in-vitro studies, which are conducted outside of living organisms. In-vitro studies, derived from the Latin term "in glass," involve experiments performed in controlled laboratory settings using cells or tissues. It is important to note that these products are not categorized as medicines or drugs, and they have not received approval from the FDA for the prevention, treatment, or cure of any medical condition, ailment, or disease. We must emphasize that any form of bodily introduction of these products into humans or animals is strictly prohibited by law. It is essential to adhere to these guidelines to ensure compliance with legal and ethical standards in research and experimentation.