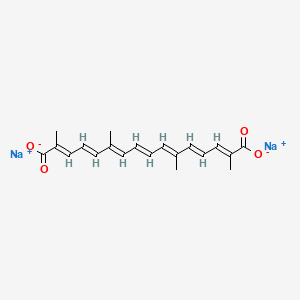
Trans sodium crocetinate
Overview
Description
Trans Sodium Crocetinate (TSC) is a synthetic carotenoid that improves the diffusion of oxygen by reducing oxygen transfer resistance within plasma . It is currently being developed to enhance oxygen delivery to hypoxic tissues in multiple conditions .
Molecular Structure Analysis
The chemical formula of TSC is C20H22Na2O4 . Its molecular weight is 372.37 . For a detailed molecular structure, a consultation with a chemical expert or a review of scientific literature would be necessary.Scientific Research Applications
COVID-19 Treatment
TSC has been investigated for its potential to improve oxygenation in COVID-19 patients experiencing hypoxemia. A study explored the use of TSC in hospitalized patients with confirmed SARS-CoV-2 infection and hypoxemia, showing that TSC was well tolerated and could reduce the time to improvement in WHO Ordinal Scale with increasing dose .
Oxygen Diffusion Enhancement
As a synthetic carotenoid, TSC acts by improving the diffusivity of oxygen in blood plasma. This unique mechanism of action makes it a promising therapeutic agent for enhancing oxygen diffusion in various tissues and organs, potentially benefiting conditions like stroke, heart attack, and hemorrhagic shock .
Radiosensitization in Cancer Therapy
TSC is being investigated as a radiosensitizer for various cancers due to its oxygen diffusion enhancement capability. By increasing oxygenation, TSC can improve the outcome of radiotherapy, making it a valuable addition to cancer treatment regimens .
Stroke Recovery
In the context of stroke, TSC’s ability to enhance oxygen diffusion can be crucial for improving patient outcomes. By facilitating better oxygen delivery to hypoxic brain tissue, TSC may help in reducing the extent of brain damage and aiding in recovery .
Hypoxia-Related Medical Emergencies
TSC is under development for treating hypoxia in patients with conditions such as cancer. Its safety profile and the compelling preclinical data suggest that TSC could be a beneficial treatment for hypoxia-related medical emergencies .
Anti-Hypoxia Potential
Research indicates that TSC can upregulate hypoxic tissue oxygenation and be effective in treating some hypoxia-related diseases. This opens up possibilities for its use in a range of conditions where tissue hypoxia is a significant concern .
Pharmacokinetics and Safety
Population pharmacokinetics analyses based on previous studies have evaluated TSC’s concentration profiles and validated two-compartment linear pharmacokinetic models. These studies also assess covariates such as body size, age, sex, organ function, and dose level .
Future Therapeutic Applications
Given the potential of TSC as a first-in-class agent, it is expected that future therapeutics based on the core concept of improved oxygen diffusion will be developed. These could satisfy the unmet medical need for many diseases and disorders, leveraging the properties of TSC .
Mechanism of Action
Target of Action
Trans Sodium Crocetinate (TSC) is a synthetic carotenoid compound . Its primary target is oxygen compounds . TSC is designed to enhance oxygenation to hypoxic (oxygen-starved) tissues .
Mode of Action
TSC works by increasing the diffusion rate of oxygen in blood plasma . Unlike most drugs that are based on biochemistry-based mechanisms, TSC’s mechanism of action is based on physical-chemical principles . It increases the movement of oxygen from red blood cells into hypoxic tissues .
Biochemical Pathways
TSC doesn’t directly interact with biochemical pathways. Instead, it enhances the diffusivity of oxygen in blood plasma . By improving oxygen diffusion, TSC can potentially alleviate conditions caused by hypoxia, such as ischemic conditions, hemorrhagic shock, and certain types of cancer .
Pharmacokinetics
It’s known that tsc is administered via intravenous bolus . More research is needed to fully understand the pharmacokinetic properties of TSC.
Result of Action
TSC has been shown to increase whole-body oxygen consumption during hemorrhagic shock . It has been suggested as a general treatment for hypoxemia . In a study involving experimental autoimmune encephalomyelitis (EAE), a model for Multiple Sclerosis (MS), TSC demonstrated anti-inflammatory, antioxidant, and anti-mitophagy effects .
Action Environment
It’s worth noting that the effectiveness of tsc may be influenced by the severity of the hypoxic condition it’s being used to treat
Future Directions
TSC is currently being developed to enhance oxygen delivery to hypoxic tissues in multiple conditions . It is under intense clinical investigation, including Phase 1b/2b clinical trials for COVID-19 . It is also being investigated for various cancers as a radiosensitizer due to its oxygen diffusion enhancement capability . Diffusion Pharmaceuticals expects to initiate a Phase 2 trial by the end of 2022 and anticipates dosing the first patient in the trial in the first quarter of 2023 .
properties
IUPAC Name |
disodium;(2E,4E,6E,8E,10E,12E,14E)-2,6,11,15-tetramethylhexadeca-2,4,6,8,10,12,14-heptaenedioate | |
---|---|---|
Source | PubChem | |
URL | https://pubchem.ncbi.nlm.nih.gov | |
Description | Data deposited in or computed by PubChem | |
InChI |
InChI=1S/C20H24O4.2Na/c1-15(11-7-13-17(3)19(21)22)9-5-6-10-16(2)12-8-14-18(4)20(23)24;;/h5-14H,1-4H3,(H,21,22)(H,23,24);;/q;2*+1/p-2/b6-5+,11-7+,12-8+,15-9+,16-10+,17-13+,18-14+;; | |
Source | PubChem | |
URL | https://pubchem.ncbi.nlm.nih.gov | |
Description | Data deposited in or computed by PubChem | |
InChI Key |
RMDMBHQVNHQDDD-VFWKRBOSSA-L | |
Source | PubChem | |
URL | https://pubchem.ncbi.nlm.nih.gov | |
Description | Data deposited in or computed by PubChem | |
Canonical SMILES |
CC(=CC=CC=C(C)C=CC=C(C)C(=O)[O-])C=CC=C(C)C(=O)[O-].[Na+].[Na+] | |
Source | PubChem | |
URL | https://pubchem.ncbi.nlm.nih.gov | |
Description | Data deposited in or computed by PubChem | |
Isomeric SMILES |
C/C(=C\C=C\C=C(\C=C\C=C(\C(=O)[O-])/C)/C)/C=C/C=C(/C(=O)[O-])\C.[Na+].[Na+] | |
Source | PubChem | |
URL | https://pubchem.ncbi.nlm.nih.gov | |
Description | Data deposited in or computed by PubChem | |
Molecular Formula |
C20H22Na2O4 | |
Source | PubChem | |
URL | https://pubchem.ncbi.nlm.nih.gov | |
Description | Data deposited in or computed by PubChem | |
Molecular Weight |
372.4 g/mol | |
Source | PubChem | |
URL | https://pubchem.ncbi.nlm.nih.gov | |
Description | Data deposited in or computed by PubChem | |
Product Name |
Trans sodium crocetinate | |
CAS RN |
591230-99-8 | |
Record name | Transcrocetinate sodium [USAN] | |
Source | ChemIDplus | |
URL | https://pubchem.ncbi.nlm.nih.gov/substance/?source=chemidplus&sourceid=0591230998 | |
Description | ChemIDplus is a free, web search system that provides access to the structure and nomenclature authority files used for the identification of chemical substances cited in National Library of Medicine (NLM) databases, including the TOXNET system. | |
Record name | TRANSCROCETINATE SODIUM | |
Source | FDA Global Substance Registration System (GSRS) | |
URL | https://gsrs.ncats.nih.gov/ginas/app/beta/substances/YP57637WMX | |
Description | The FDA Global Substance Registration System (GSRS) enables the efficient and accurate exchange of information on what substances are in regulated products. Instead of relying on names, which vary across regulatory domains, countries, and regions, the GSRS knowledge base makes it possible for substances to be defined by standardized, scientific descriptions. | |
Explanation | Unless otherwise noted, the contents of the FDA website (www.fda.gov), both text and graphics, are not copyrighted. They are in the public domain and may be republished, reprinted and otherwise used freely by anyone without the need to obtain permission from FDA. Credit to the U.S. Food and Drug Administration as the source is appreciated but not required. | |
Retrosynthesis Analysis
AI-Powered Synthesis Planning: Our tool employs the Template_relevance Pistachio, Template_relevance Bkms_metabolic, Template_relevance Pistachio_ringbreaker, Template_relevance Reaxys, Template_relevance Reaxys_biocatalysis model, leveraging a vast database of chemical reactions to predict feasible synthetic routes.
One-Step Synthesis Focus: Specifically designed for one-step synthesis, it provides concise and direct routes for your target compounds, streamlining the synthesis process.
Accurate Predictions: Utilizing the extensive PISTACHIO, BKMS_METABOLIC, PISTACHIO_RINGBREAKER, REAXYS, REAXYS_BIOCATALYSIS database, our tool offers high-accuracy predictions, reflecting the latest in chemical research and data.
Strategy Settings
Precursor scoring | Relevance Heuristic |
---|---|
Min. plausibility | 0.01 |
Model | Template_relevance |
Template Set | Pistachio/Bkms_metabolic/Pistachio_ringbreaker/Reaxys/Reaxys_biocatalysis |
Top-N result to add to graph | 6 |
Feasible Synthetic Routes
Disclaimer and Information on In-Vitro Research Products
Please be aware that all articles and product information presented on BenchChem are intended solely for informational purposes. The products available for purchase on BenchChem are specifically designed for in-vitro studies, which are conducted outside of living organisms. In-vitro studies, derived from the Latin term "in glass," involve experiments performed in controlled laboratory settings using cells or tissues. It is important to note that these products are not categorized as medicines or drugs, and they have not received approval from the FDA for the prevention, treatment, or cure of any medical condition, ailment, or disease. We must emphasize that any form of bodily introduction of these products into humans or animals is strictly prohibited by law. It is essential to adhere to these guidelines to ensure compliance with legal and ethical standards in research and experimentation.