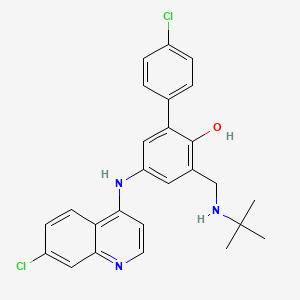
Tebuquine
- Click on QUICK INQUIRY to receive a quote from our team of experts.
- With the quality product at a COMPETITIVE price, you can focus more on your research.
Overview
Description
- Tebuquine is a synthetic compound that does not occur naturally. It is primarily used as an antimalarial drug.
- Technically, this compound is part of the human exposome , which encompasses all the exposures an individual encounters throughout their lifetime and how those exposures relate to health .
Preparation Methods
- Tebuquine can be synthesized through various routes. One common method involves starting from p-chloroiodobenzene as a raw material.
- The synthetic process typically includes cyclization reactions and functional group transformations to yield this compound.
- Industrial production methods may involve large-scale synthesis using optimized conditions.
Chemical Reactions Analysis
- Tebuquine undergoes several types of reactions, including oxidation , reduction , and substitution .
- Common reagents used in these reactions include oxidizing agents (e.g., chromium trioxide ), reducing agents (e.g., sodium borohydride ), and nucleophiles (e.g., amines ).
- Major products formed from these reactions include various derivatives of this compound.
Scientific Research Applications
- Tebuquine has applications beyond antimalarial use:
Chemistry: It serves as a valuable synthetic intermediate for other compounds.
Biology: Researchers study its effects on cellular processes and potential therapeutic applications.
Medicine: Besides antimalarial properties, it may have applications in treating other diseases.
Industry: this compound’s derivatives find use in the pharmaceutical and agrochemical sectors.
Mechanism of Action
- Tebuquine’s antimalarial activity involves targeting the Plasmodium parasite.
- It interferes with heme detoxification, disrupting the parasite’s ability to neutralize toxic heme by converting it into hemozoin.
- Molecular targets include heme polymerase and hemozoin formation pathways.
Comparison with Similar Compounds
- Tebuquine shares similarities with other antimalarial drugs, such as chloroquine and quinine .
- Its uniqueness lies in its improved efficacy against chloroquine-resistant strains of Plasmodium falciparum .
Properties
CAS No. |
74129-03-6 |
---|---|
Molecular Formula |
C26H25Cl2N3O |
Molecular Weight |
466.4 g/mol |
IUPAC Name |
2-[(tert-butylamino)methyl]-6-(4-chlorophenyl)-4-[(7-chloroquinolin-4-yl)amino]phenol |
InChI |
InChI=1S/C26H25Cl2N3O/c1-26(2,3)30-15-17-12-20(14-22(25(17)32)16-4-6-18(27)7-5-16)31-23-10-11-29-24-13-19(28)8-9-21(23)24/h4-14,30,32H,15H2,1-3H3,(H,29,31) |
InChI Key |
BCHMRNALCJISMZ-UHFFFAOYSA-N |
SMILES |
CC(C)(C)NCC1=C(C(=CC(=C1)NC2=C3C=CC(=CC3=NC=C2)Cl)C4=CC=C(C=C4)Cl)O |
Canonical SMILES |
CC(C)(C)NCC1=C(C(=CC(=C1)NC2=C3C=CC(=CC3=NC=C2)Cl)C4=CC=C(C=C4)Cl)O |
Appearance |
Solid powder |
Key on ui other cas no. |
74129-03-6 |
Purity |
>98% (or refer to the Certificate of Analysis) |
shelf_life |
>2 years if stored properly |
solubility |
Soluble in DMSO |
storage |
Dry, dark and at 0 - 4 C for short term (days to weeks) or -20 C for long term (months to years). |
Synonyms |
Tebuquine; CI-897; CI897; CI 897; WR-228,258; WR 228,258; WR228,258; WR-228258; WR 228258; WR228258. |
Origin of Product |
United States |
Retrosynthesis Analysis
AI-Powered Synthesis Planning: Our tool employs the Template_relevance Pistachio, Template_relevance Bkms_metabolic, Template_relevance Pistachio_ringbreaker, Template_relevance Reaxys, Template_relevance Reaxys_biocatalysis model, leveraging a vast database of chemical reactions to predict feasible synthetic routes.
One-Step Synthesis Focus: Specifically designed for one-step synthesis, it provides concise and direct routes for your target compounds, streamlining the synthesis process.
Accurate Predictions: Utilizing the extensive PISTACHIO, BKMS_METABOLIC, PISTACHIO_RINGBREAKER, REAXYS, REAXYS_BIOCATALYSIS database, our tool offers high-accuracy predictions, reflecting the latest in chemical research and data.
Strategy Settings
Precursor scoring | Relevance Heuristic |
---|---|
Min. plausibility | 0.01 |
Model | Template_relevance |
Template Set | Pistachio/Bkms_metabolic/Pistachio_ringbreaker/Reaxys/Reaxys_biocatalysis |
Top-N result to add to graph | 6 |
Feasible Synthetic Routes
Disclaimer and Information on In-Vitro Research Products
Please be aware that all articles and product information presented on BenchChem are intended solely for informational purposes. The products available for purchase on BenchChem are specifically designed for in-vitro studies, which are conducted outside of living organisms. In-vitro studies, derived from the Latin term "in glass," involve experiments performed in controlled laboratory settings using cells or tissues. It is important to note that these products are not categorized as medicines or drugs, and they have not received approval from the FDA for the prevention, treatment, or cure of any medical condition, ailment, or disease. We must emphasize that any form of bodily introduction of these products into humans or animals is strictly prohibited by law. It is essential to adhere to these guidelines to ensure compliance with legal and ethical standards in research and experimentation.