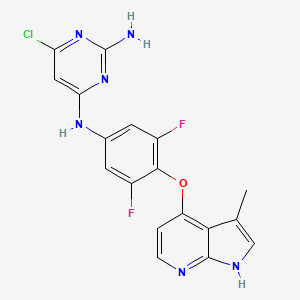
ROCK Inhibitor
Overview
Description
BAY-549, also known as Azaindole 1, is a highly potent and selective inhibitor of Rho-associated protein kinase 1 and Rho-associated protein kinase 2. These kinases are involved in various cellular functions, including contraction, motility, proliferation, and apoptosis. BAY-549 is an orally active and ATP-competitive inhibitor with significant potential in scientific research and therapeutic applications .
Preparation Methods
Synthetic Routes and Reaction Conditions: The synthesis of BAY-549 involves multiple steps, starting with the preparation of the azaindole core. The key steps include:
- Formation of the azaindole ring through cyclization reactions.
- Introduction of the chloro and difluorophenyl groups via substitution reactions.
- Final purification to achieve high purity levels.
Industrial Production Methods: Industrial production of BAY-549 follows similar synthetic routes but on a larger scale. The process involves:
- Optimization of reaction conditions to maximize yield and purity.
- Use of industrial-grade reagents and solvents.
- Implementation of stringent quality control measures to ensure consistency and safety .
Chemical Reactions Analysis
Types of Reactions: BAY-549 undergoes various chemical reactions, including:
Oxidation: Involves the addition of oxygen or removal of hydrogen.
Reduction: Involves the addition of hydrogen or removal of oxygen.
Substitution: Involves the replacement of one functional group with another.
Common Reagents and Conditions:
Oxidation: Common reagents include potassium permanganate and hydrogen peroxide.
Reduction: Common reagents include lithium aluminum hydride and sodium borohydride.
Substitution: Common reagents include halogens and nucleophiles.
Major Products: The major products formed from these reactions depend on the specific conditions and reagents used. For example, oxidation may lead to the formation of hydroxylated derivatives, while substitution reactions may yield various substituted azaindole derivatives .
Scientific Research Applications
BAY-549 has a wide range of scientific research applications, including:
Chemistry: Used as a chemical probe to study the inhibition of Rho-associated protein kinase 1 and Rho-associated protein kinase 2.
Biology: Investigates the role of Rho-associated protein kinase in cellular functions such as motility and apoptosis.
Medicine: Explores potential therapeutic applications in treating cardiovascular diseases, hypertension, and other conditions.
Industry: Utilized in the development of new drugs and therapeutic agents
Mechanism of Action
BAY-549 exerts its effects by inhibiting the activity of Rho-associated protein kinase 1 and Rho-associated protein kinase 2. These kinases play a crucial role in regulating the cytoskeleton, cell shape, and motility. By inhibiting these kinases, BAY-549 can modulate various cellular processes, leading to potential therapeutic benefits. The molecular targets and pathways involved include the Rho/ROCK signaling pathway, which is essential for various cellular functions .
Comparison with Similar Compounds
Fasudil: Another Rho-associated protein kinase inhibitor with similar therapeutic applications.
Y-27632: A selective inhibitor of Rho-associated protein kinase with applications in research and therapy.
Comparison:
Potency: BAY-549 has higher potency compared to Fasudil and Y-27632, with lower IC50 values for Rho-associated protein kinase 1 and Rho-associated protein kinase 2.
Selectivity: BAY-549 exhibits greater selectivity for Rho-associated protein kinase 1 and Rho-associated protein kinase 2, reducing off-target effects.
Oral Activity: BAY-549 is orally active, making it more convenient for therapeutic use compared to some other inhibitors .
Properties
IUPAC Name |
6-chloro-4-N-[3,5-difluoro-4-[(3-methyl-1H-pyrrolo[2,3-b]pyridin-4-yl)oxy]phenyl]pyrimidine-2,4-diamine | |
---|---|---|
Source | PubChem | |
URL | https://pubchem.ncbi.nlm.nih.gov | |
Description | Data deposited in or computed by PubChem | |
InChI |
InChI=1S/C18H13ClF2N6O/c1-8-7-24-17-15(8)12(2-3-23-17)28-16-10(20)4-9(5-11(16)21)25-14-6-13(19)26-18(22)27-14/h2-7H,1H3,(H,23,24)(H3,22,25,26,27) | |
Source | PubChem | |
URL | https://pubchem.ncbi.nlm.nih.gov | |
Description | Data deposited in or computed by PubChem | |
InChI Key |
NRSGWEVTVGZDFC-UHFFFAOYSA-N | |
Source | PubChem | |
URL | https://pubchem.ncbi.nlm.nih.gov | |
Description | Data deposited in or computed by PubChem | |
Canonical SMILES |
CC1=CNC2=NC=CC(=C12)OC3=C(C=C(C=C3F)NC4=CC(=NC(=N4)N)Cl)F | |
Source | PubChem | |
URL | https://pubchem.ncbi.nlm.nih.gov | |
Description | Data deposited in or computed by PubChem | |
Molecular Formula |
C18H13ClF2N6O | |
Source | PubChem | |
URL | https://pubchem.ncbi.nlm.nih.gov | |
Description | Data deposited in or computed by PubChem | |
DSSTOX Substance ID |
DTXSID00468040 | |
Record name | TC-S 7001 | |
Source | EPA DSSTox | |
URL | https://comptox.epa.gov/dashboard/DTXSID00468040 | |
Description | DSSTox provides a high quality public chemistry resource for supporting improved predictive toxicology. | |
Molecular Weight |
402.8 g/mol | |
Source | PubChem | |
URL | https://pubchem.ncbi.nlm.nih.gov | |
Description | Data deposited in or computed by PubChem | |
CAS No. |
867017-68-3 | |
Record name | TC-S 7001 | |
Source | EPA DSSTox | |
URL | https://comptox.epa.gov/dashboard/DTXSID00468040 | |
Description | DSSTox provides a high quality public chemistry resource for supporting improved predictive toxicology. | |
Synthesis routes and methods
Procedure details
Retrosynthesis Analysis
AI-Powered Synthesis Planning: Our tool employs the Template_relevance Pistachio, Template_relevance Bkms_metabolic, Template_relevance Pistachio_ringbreaker, Template_relevance Reaxys, Template_relevance Reaxys_biocatalysis model, leveraging a vast database of chemical reactions to predict feasible synthetic routes.
One-Step Synthesis Focus: Specifically designed for one-step synthesis, it provides concise and direct routes for your target compounds, streamlining the synthesis process.
Accurate Predictions: Utilizing the extensive PISTACHIO, BKMS_METABOLIC, PISTACHIO_RINGBREAKER, REAXYS, REAXYS_BIOCATALYSIS database, our tool offers high-accuracy predictions, reflecting the latest in chemical research and data.
Strategy Settings
Precursor scoring | Relevance Heuristic |
---|---|
Min. plausibility | 0.01 |
Model | Template_relevance |
Template Set | Pistachio/Bkms_metabolic/Pistachio_ringbreaker/Reaxys/Reaxys_biocatalysis |
Top-N result to add to graph | 6 |
Feasible Synthetic Routes
Q1: What are ROCKs and what is their role in cellular processes?
A1: ROCKs are serine/threonine protein kinases that act as downstream effectors of the small GTPase RhoA. [] They play a crucial role in various cellular functions, including:
- Smooth muscle contraction: ROCK phosphorylates the myosin-binding subunit of myosin light chain (MLC) phosphatase, inhibiting its activity and leading to contraction. []
- Cell adhesion and migration: ROCK regulates actin cytoskeletal reorganization, impacting cell adhesion, migration, and morphology. []
- Apoptosis and inflammation: ROCK signaling influences apoptosis, inflammation, and other cellular responses. [, ]
Q2: How do ROCK inhibitors exert their therapeutic effects?
A2: ROCK inhibitors bind to and inhibit the activity of ROCK kinases, thereby modulating their downstream effects. This inhibition can lead to:
- Reduced smooth muscle contraction: Beneficial in conditions like hypertension and asthma by promoting vasodilation and bronchodilation. [, ]
- Enhanced corneal wound healing: ROCK inhibitors promote corneal endothelial cell migration and proliferation, aiding in wound healing. [, ]
- Suppressed fibrosis: ROCK inhibition can reduce the activation of fibroblasts and the synthesis of extracellular matrix proteins, ameliorating fibrosis in organs like the liver and lungs. [, ]
Q3: Is there a difference between the effects of ROCK1 and ROCK2 inhibition?
A3: While both ROCK1 and ROCK2 are involved in various cellular processes, emerging evidence suggests distinct roles for each isoform. For instance, ROCK1 inhibition was found to be selectively lethal to von Hippel-Lindau (VHL)-deficient clear cell renal cell carcinoma (CC-RCC), while ROCK2 knockdown had no effect. [] Additionally, selective inhibition of ROCK1 using the compound GSK429286 showed potent pro-angiogenic properties and enhanced blood flow recovery in a mouse model of hindlimb ischemia. []
Q4: What are some examples of ROCK inhibitors and their structural characteristics?
A4: Several ROCK inhibitors have been developed, each with unique structural features. Some notable examples include:
- Fasudil [(S)-(+)-(2-methyl-5-isoquinolinyl) sulfonylhomopiperazine, 2HCl]: A first-generation ROCK inhibitor used clinically for cerebral vasospasm. [, ]
- Y-27632 [(R)-(+)-trans-N-(4-pyridyl)-4-(1-aminoethyl)-cyclohexanecarbox anecarboxamide, 2HCl]: A widely used this compound in research, known for its role in promoting stem cell survival and proliferation. [, ]
- Ripasudil: A fasudil derivative approved for clinical use in glaucoma and ocular hypertension. []
- GSK429286: An indazole-based this compound demonstrating high selectivity for ROCK1 and potent pro-angiogenic effects. []
- AT13148: An AGC kinase inhibitor targeting both ROCK1/2 and S6 kinase, showing potent synthetic lethality in VHL-deficient CC-RCC. []
Q5: Can you provide more information on the chemical structure and properties of these inhibitors?
A5: Unfortunately, the provided research abstracts do not include detailed information regarding the molecular formula, weight, or spectroscopic data for each this compound. Accessing comprehensive chemical databases and scientific literature on these specific compounds would be necessary for a more in-depth structural understanding.
Q6: How do structural modifications of ROCK inhibitors affect their activity and selectivity?
A6: Structural modifications significantly influence the potency and selectivity of ROCK inhibitors. For instance, fasudil and ripasudil, both isoquinoline derivatives, exhibit different pharmacological properties due to variations in their substituent groups. [] Similarly, the indazole-based GSK429286 demonstrates enhanced selectivity for ROCK isoforms compared to other ROCK inhibitors like fasudil. []
Q7: Are there efforts to develop ROCK inhibitors with improved selectivity profiles?
A7: Yes, researchers are actively exploring structural modifications to develop ROCK inhibitors with enhanced selectivity for specific ROCK isoforms or downstream targets. This selectivity is crucial for minimizing potential side effects and enhancing therapeutic efficacy in various diseases. [, , ]
Disclaimer and Information on In-Vitro Research Products
Please be aware that all articles and product information presented on BenchChem are intended solely for informational purposes. The products available for purchase on BenchChem are specifically designed for in-vitro studies, which are conducted outside of living organisms. In-vitro studies, derived from the Latin term "in glass," involve experiments performed in controlled laboratory settings using cells or tissues. It is important to note that these products are not categorized as medicines or drugs, and they have not received approval from the FDA for the prevention, treatment, or cure of any medical condition, ailment, or disease. We must emphasize that any form of bodily introduction of these products into humans or animals is strictly prohibited by law. It is essential to adhere to these guidelines to ensure compliance with legal and ethical standards in research and experimentation.