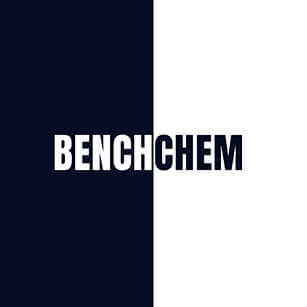
Swertianolin
Overview
Description
Swertianolin is a xanthone glycoside compound isolated from various species of the Swertia genus, particularly from Swertia japonica and Gentiana campestris . It is known for its diverse biological activities, including anti-Helicobacter pylori, antioxidant, and acetylcholinesterase inhibitory properties .
Mechanism of Action
Target of Action
Swertianolin, a xanthone isolated from Gentianella Acuta, primarily targets Helicobacter pylori , a Gram-negative, spiral-shaped, motile bacterium present in human stomachs . This bacterium is associated with gastric disorders such as chronic gastritis, duodenal ulcer, and gastric cancer . This compound also inhibits acetylcholinesterase (AChE) .
Mode of Action
This compound interacts with its targets to exert its therapeutic effects. It has demonstrated significant anti-Helicobacter pylori activity . The compound also inhibits acetylcholinesterase (AChE), an enzyme that breaks down acetylcholine, a neurotransmitter in the nervous system .
Biochemical Pathways
The biosynthesis of this compound involves the shikimate pathway, either through L-phenylalanine-dependent or -independent pathway, that later forms an intermediate benzophenone, 2,3′,4,6-tetrahydoxybenzophenone . This is followed by a regioselective intramolecular mediated oxidative coupling to form xanthone ring compounds .
Pharmacokinetics
A sensitive and rapid LC-MS/MS method has been developed and validated for quantifying this compound in rat plasma . The lower limit of quantitation was 0.5ng/mL within a linear range of 0.5-500ng/mL . The mean recovery of this compound was >66.7% . The method was successfully applied in evaluating the pharmacokinetics of this compound after an oral dose of 50mg/kg Swertia mussotii extract in rats .
Biochemical Analysis
Biochemical Properties
Swertianolin plays a significant role in biochemical reactions due to its interaction with various enzymes, proteins, and other biomolecules. One of the key interactions is with acetylcholinesterase, an enzyme responsible for breaking down the neurotransmitter acetylcholine. This compound exhibits anti-acetylcholinesterase activity, which can enhance cholinergic transmission by inhibiting the breakdown of acetylcholine . Additionally, this compound interacts with antioxidant enzymes, contributing to its ability to scavenge free radicals and reduce oxidative stress . These interactions highlight the compound’s potential in modulating enzymatic activities and protecting cells from oxidative damage.
Cellular Effects
This compound exerts various effects on different types of cells and cellular processes. In neuronal cells, this compound’s anti-acetylcholinesterase activity can enhance cholinergic signaling, potentially improving cognitive functions and offering therapeutic benefits for neurodegenerative diseases . In addition, this compound’s antioxidant properties help protect cells from oxidative stress-induced damage, which is crucial for maintaining cellular homeostasis . The compound also influences cell signaling pathways, gene expression, and cellular metabolism, contributing to its diverse biological activities.
Molecular Mechanism
The molecular mechanism of this compound involves its binding interactions with biomolecules, enzyme inhibition, and changes in gene expression. This compound binds to the active site of acetylcholinesterase, inhibiting its activity and preventing the breakdown of acetylcholine . This inhibition enhances cholinergic transmission and can improve cognitive functions. Furthermore, this compound’s antioxidant activity is mediated through its interaction with reactive oxygen species and antioxidant enzymes, reducing oxidative stress and protecting cells from damage . These molecular interactions underline the compound’s therapeutic potential in various biological processes.
Temporal Effects in Laboratory Settings
In laboratory settings, the effects of this compound can change over time due to its stability, degradation, and long-term impact on cellular function. Studies have shown that this compound remains stable under controlled conditions, maintaining its biological activity . Prolonged exposure to environmental factors such as light and temperature can lead to its degradation, reducing its efficacy. Long-term studies in vitro and in vivo have demonstrated that this compound can sustain its protective effects on cells, highlighting its potential for therapeutic applications .
Dosage Effects in Animal Models
The effects of this compound vary with different dosages in animal models. At lower doses, this compound exhibits beneficial effects such as enhanced cognitive function and reduced oxidative stress . At higher doses, the compound may exhibit toxic or adverse effects, including hepatotoxicity and gastrointestinal disturbances . These threshold effects emphasize the importance of determining the optimal dosage for therapeutic applications to maximize benefits while minimizing risks.
Metabolic Pathways
This compound is involved in various metabolic pathways, interacting with enzymes and cofactors that influence its metabolism. The compound undergoes biotransformation in the liver, where it is metabolized by cytochrome P450 enzymes . This metabolism can lead to the formation of active or inactive metabolites, affecting its biological activity. This compound also influences metabolic flux and metabolite levels, contributing to its diverse biological effects.
Transport and Distribution
This compound is transported and distributed within cells and tissues through interactions with transporters and binding proteins. The compound can cross cellular membranes and accumulate in specific tissues, influencing its localization and activity . Transporters such as organic anion-transporting polypeptides (OATPs) and multidrug resistance-associated proteins (MRPs) play a role in this compound’s cellular uptake and distribution . These interactions determine the compound’s bioavailability and therapeutic potential.
Subcellular Localization
The subcellular localization of this compound affects its activity and function. The compound can be directed to specific compartments or organelles through targeting signals and post-translational modifications . For example, this compound may localize to the mitochondria, where it exerts its antioxidant effects by scavenging reactive oxygen species and protecting mitochondrial function . Understanding the subcellular localization of this compound provides insights into its mechanism of action and potential therapeutic applications.
Preparation Methods
Swertianolin can be extracted from the Swertia herb using a methanolic extract. The dried extract is dissolved in water and partitioned with n-hexane, ethyl acetate, and n-butanol successively. The part soluble in ethyl acetate shows effective anti-Helicobacter pylori activity, and this compound can be isolated from this fraction
Chemical Reactions Analysis
Swertianolin undergoes various chemical reactions, including:
Oxidation: This compound acts as an antioxidant, opposing oxidation reactions brought about by dioxygen or peroxides.
Substitution: The xanthone nucleus of this compound can undergo substitution reactions, particularly at the hydroxyl and methoxy positions.
Common reagents and conditions used in these reactions include oxidizing agents for oxidation reactions and various nucleophiles for substitution reactions. The major products formed from these reactions depend on the specific conditions and reagents used.
Scientific Research Applications
Swertianolin has several scientific research applications:
Comparison with Similar Compounds
Swertianolin is unique due to its combination of antioxidant, anti-Helicobacter pylori, and acetylcholinesterase inhibitory properties. Similar compounds include:
Properties
CAS No. |
23445-00-3 |
---|---|
Molecular Formula |
C20H20O11 |
Molecular Weight |
436.4 g/mol |
IUPAC Name |
1,5-dihydroxy-3-methoxy-8-[(2S,3R,4S,5S,6R)-3,4,5-trihydroxy-6-(hydroxymethyl)oxan-2-yl]oxyxanthen-9-one |
InChI |
InChI=1S/C20H20O11/c1-28-7-4-9(23)13-11(5-7)29-19-8(22)2-3-10(14(19)16(13)25)30-20-18(27)17(26)15(24)12(6-21)31-20/h2-5,12,15,17-18,20-24,26-27H,6H2,1H3/t12-,15-,17+,18-,20-/m1/s1 |
InChI Key |
XMVBNLMKPMPWAX-DIKOWXHZSA-N |
Isomeric SMILES |
COC1=CC(=C2C(=C1)OC3=C(C=CC(=C3C2=O)O[C@H]4[C@@H]([C@H]([C@@H]([C@H](O4)CO)O)O)O)O)O |
SMILES |
O=C1C2=C(OC3=C1C(O)=CC=C3O)C=C(OC)C=C2O[C@H]4[C@@H]([C@H]([C@@H]([C@@H](CO)O4)O)O)O |
Canonical SMILES |
COC1=CC(=C2C(=C1)OC3=C(C=CC(=C3C2=O)OC4C(C(C(C(O4)CO)O)O)O)O)O |
Appearance |
Solid powder |
Purity |
>98% (or refer to the Certificate of Analysis) |
shelf_life |
>2 years if stored properly |
solubility |
Soluble in DMSO |
storage |
Dry, dark and at 0 - 4 C for short term (days to weeks) or -20 C for long term (months to years). |
Synonyms |
Swertianolin; CCRIS 5474; CCRIS-5474 CCRIS5474. |
Origin of Product |
United States |
Retrosynthesis Analysis
AI-Powered Synthesis Planning: Our tool employs the Template_relevance Pistachio, Template_relevance Bkms_metabolic, Template_relevance Pistachio_ringbreaker, Template_relevance Reaxys, Template_relevance Reaxys_biocatalysis model, leveraging a vast database of chemical reactions to predict feasible synthetic routes.
One-Step Synthesis Focus: Specifically designed for one-step synthesis, it provides concise and direct routes for your target compounds, streamlining the synthesis process.
Accurate Predictions: Utilizing the extensive PISTACHIO, BKMS_METABOLIC, PISTACHIO_RINGBREAKER, REAXYS, REAXYS_BIOCATALYSIS database, our tool offers high-accuracy predictions, reflecting the latest in chemical research and data.
Strategy Settings
Precursor scoring | Relevance Heuristic |
---|---|
Min. plausibility | 0.01 |
Model | Template_relevance |
Template Set | Pistachio/Bkms_metabolic/Pistachio_ringbreaker/Reaxys/Reaxys_biocatalysis |
Top-N result to add to graph | 6 |
Feasible Synthetic Routes
Q1: What is the primary mechanism of action for swertianolin in the context of sepsis?
A1: this compound has been shown to ameliorate immune dysfunction in sepsis by targeting myeloid-derived suppressor cells (MDSCs). It achieves this by blocking the immunosuppressive function of MDSCs, reducing their production of interleukin-10 (IL-10), nitric oxide (NO), reactive oxygen species (ROS), and arginase. []
Q2: How does this compound impact MDSC proliferation and differentiation?
A2: this compound has been found to reduce MDSC proliferation and promote their differentiation into dendritic cells, thereby counteracting the immunosuppressive environment often seen in sepsis. []
Q3: Does this compound directly affect T-cell activity?
A3: While this compound doesn't directly act on T-cells, its ability to block the immunosuppressive effects of MDSCs indirectly leads to improved T-cell activity. []
Q4: What is the significance of the different MDSC subtypes (G-MDSCs and M-MDSCs) in sepsis, and how does this compound affect them?
A4: Both G-MDSCs and M-MDSCs significantly increase in the bone marrow and spleen during sepsis, with G-MDSCs being the predominant subtype in a murine model of polymicrobial peritonitis. This compound effectively regulates the functions of both MDSC subsets, reducing their immunosuppressive effects. []
Q5: Has this compound demonstrated activity against any specific diseases?
A5: Research suggests that this compound exhibits anti-HBV activity in vitro, showing potential for suppressing Hepatitis B surface antigen (HBsAg) and e antigen (HBeAg) expression in cultured human hepatocellular carcinoma cell lines (HepG2). [] Additionally, this compound demonstrated potential neuroprotective effects against 1-methyl-4-phenylpyridinium-induced apoptosis in the SH-SY5Y human neuroblastoma cell line. [] Furthermore, this compound exhibited Anti-Helicobacter pylori activity. []
Q6: What enzymes does this compound interact with?
A6: this compound has been identified as a potential α-glucosidase inhibitor. [] Research also indicates that it exhibits inhibitory activity against monoamine oxidase B (MAO B). []
Q7: What is the molecular formula and weight of this compound?
A7: this compound has a molecular formula of C20H20O11 and a molecular weight of 436.37 g/mol. [, ]
Q8: What is the chemical structure of this compound?
A8: this compound is a xanthone glycoside. It consists of a xanthone nucleus (1,3,5,8-tetrahydroxyxanthone) with a glucose moiety attached at the 8-position via an O-glycosidic bond. []
Q9: What spectroscopic techniques have been used to characterize this compound?
A9: Various spectroscopic methods have been employed to elucidate the structure of this compound, including UV-Vis spectroscopy, IR spectroscopy, mass spectrometry (MS), and nuclear magnetic resonance (NMR) spectroscopy, including both 1D (1H and 13C) and 2D NMR techniques. [, , , , , , ]
Q10: What is the significance of the revised structure of this compound?
A11: The revised structure accurately reflects the connectivity of the glucose moiety to the xanthone nucleus. This is essential for understanding its physicochemical properties, biological activity, and potential for synthesis and modification. []
Q11: Are there any studies on the stability of this compound under various conditions?
A12: While the provided research abstracts do not delve into specific stability studies for this compound, they emphasize the importance of analytical method validation for quality control during development, manufacturing, and distribution. This suggests a focus on ensuring the compound's consistency, safety, and efficacy, which indirectly points to stability considerations. []
Q12: What is known about the pharmacokinetics of this compound?
A13: A sensitive and rapid LC-MS/MS method has been developed and validated for quantifying this compound in rat plasma using rutin as an internal standard, and successfully applied to evaluate the pharmacokinetics of this compound after an oral dose of 50 mg/kg Swertia mussotii extract in rats. []
Q13: What analytical methods are commonly used for the detection and quantification of this compound?
A14: Several analytical techniques have been employed for this compound analysis, with a prominent focus on high-performance liquid chromatography (HPLC). Researchers have utilized various HPLC methods, including RP-HPLC (Reverse Phase HPLC) and HPLC coupled with multi-wavelength detection. These methods have proven effective for both qualitative and quantitative analysis of this compound in various plant materials and extracts. [, , , , , , , ]
Q14: What other methods, besides HPLC, have been used to study this compound?
A15: Beyond HPLC, researchers have employed techniques like thin-layer chromatography (TLC) for qualitative identification of this compound. Furthermore, electrospray ionization tandem mass spectrometry (ESI-MS/MS) has been utilized to investigate the structure and fragmentation mechanisms of this compound complexes with metal ions like Mn(II), Al(III), and Cu(II). These studies provide insights into the coordination behavior of this compound and its potential interactions with metal ions in biological systems. [, , ]
Q15: What is the primary natural source of this compound?
A16: this compound is predominantly found in plants belonging to the genus Swertia, which are known for their bitter taste and medicinal properties. [, , , , , , , , ]
Q16: What other plant species, besides Swertia, contain this compound?
A17: this compound has also been identified in other plant species, including: * Gentianella amarella ssp. acuta [] * Gentianopsis paludosa [, , ] * Swertia pubescens [] * Gentianella azurea [, ] * Lomatogonium rotatum [, ] * Phaleria macrocarpa []
Q17: How is this compound relevant to chemotaxonomic studies?
A18: The presence and distribution of this compound, along with other characteristic metabolites like iridoid glycosides and phenols, are valuable for chemotaxonomic studies within the Gentianaceae family. These compounds serve as potential chemotaxonomic markers to differentiate various Gentianaceae species and explore their evolutionary relationships. [, ]
Disclaimer and Information on In-Vitro Research Products
Please be aware that all articles and product information presented on BenchChem are intended solely for informational purposes. The products available for purchase on BenchChem are specifically designed for in-vitro studies, which are conducted outside of living organisms. In-vitro studies, derived from the Latin term "in glass," involve experiments performed in controlled laboratory settings using cells or tissues. It is important to note that these products are not categorized as medicines or drugs, and they have not received approval from the FDA for the prevention, treatment, or cure of any medical condition, ailment, or disease. We must emphasize that any form of bodily introduction of these products into humans or animals is strictly prohibited by law. It is essential to adhere to these guidelines to ensure compliance with legal and ethical standards in research and experimentation.