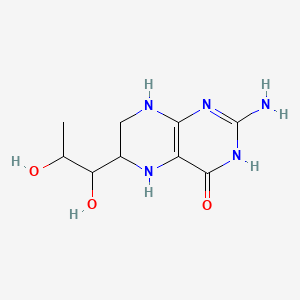
Tetrahydrobiopterin
Overview
Description
Tetrahydrobiopterin is a naturally occurring compound that serves as a cofactor for several enzymes involved in the metabolism of amino acids and the biosynthesis of neurotransmitters. It plays a crucial role in the degradation of phenylalanine and the synthesis of serotonin, melatonin, dopamine, norepinephrine, and epinephrine . Its chemical structure is that of a reduced pteridine derivative.
Mechanism of Action
Target of Action
Tetrahydrobiopterin (BH4) is a multifunctional co-factor of various enzymes . Its primary targets include the three aromatic amino acid hydroxylase enzymes . These enzymes play a crucial role in the degradation of the amino acid phenylalanine and in the biosynthesis of neurotransmitters such as serotonin, melatonin, dopamine, norepinephrine, and epinephrine . BH4 is also a co-factor for the production of nitric oxide by the nitric oxide synthases .
Mode of Action
BH4 interacts with its targets to facilitate essential biochemical conversions. For instance, when used as a co-factor, BH4 grabs molecular oxygen and attaches one oxygen atom to the target molecule . It ends up having the other oxygen attached to itself in the process . Two enzymes are then needed to strip off this unwanted oxygen and restore the co-factor . As a co-factor for phenylalanine hydroxylase (PAH), BH4 allows the conversion of phenylalanine to tyrosine .
Biochemical Pathways
BH4 is involved in several biochemical pathways. It is formed de novo from GTP via a sequence of three enzymatic steps carried out by GTP cyclohydrolase I, 6-pyruvoyltetrahydropterin synthase, and sepiapterin reductase . An alternative or salvage pathway involves dihydrofolate reductase and may play an essential role in peripheral tissues . BH4 is also essential for the metabolism of phenylalanine and lipid esters .
Pharmacokinetics
The pharmacokinetics of BH4 shows high intra-individual and inter-individual variability . After oral administration, maximum BH4 concentrations are reached 4 hours post-administration . After 24 hours, BH4 blood concentrations drop to 11% of maximal values . The pharmacokinetic parameters calculated for BH4 in blood include a half-life for absorption (1.1 hours), distribution (2.5 hours), and elimination (46.0 hours) phases .
Result of Action
The action of BH4 results in significant molecular and cellular effects. It supports essential pathways to generate energy, enhance the antioxidant resistance of cells against stressful conditions, and protect from sustained inflammation . The imbalance of BH4 may affect neurotransmitter production, leading to many abnormalities in the central nervous system .
Action Environment
The action, efficacy, and stability of BH4 can be influenced by various environmental factors. For instance, the outcome of the BH4 loading test depends on the genotype and dosage of BH4 . Moreover, environmental stressors can influence the antioxidant resistance of cells, which BH4 helps to enhance .
Biochemical Analysis
Biochemical Properties
Tetrahydrobiopterin is involved in the hydroxylation of aromatic amino acids, functioning as a cofactor for aromatic amino acid hydroxylases . It also plays a role in the synthesis of nitric oxide by acting as a cofactor for nitric oxide synthase isoenzymes .
Cellular Effects
This compound influences cell function through its role in the synthesis of monoamine neurotransmitters and nitric oxide . It is involved in cell signaling pathways related to these molecules and can influence gene expression and cellular metabolism through these pathways .
Molecular Mechanism
At the molecular level, this compound exerts its effects through its role as a cofactor for various enzymes. It is involved in binding interactions with these enzymes, potentially influencing their activity . Changes in gene expression may also occur as a result of the pathways that this compound is involved in .
Metabolic Pathways
This compound is involved in several metabolic pathways. It is formed de novo from GTP via a sequence of three enzymatic steps carried out by GTP cyclohydrolase I, 6-pyruvoyltetrahydropterin synthase, and sepiapterin reductase . It can also interact with other enzymes and cofactors in these pathways .
Preparation Methods
Synthetic Routes and Reaction Conditions: Tetrahydrobiopterin is synthesized from guanosine triphosphate through a series of enzymatic reactions. The key enzymes involved are guanosine triphosphate cyclohydrolase I, 6-pyruvoyltetrahydropterin synthase, and sepiapterin reductase . The synthetic route involves the conversion of guanosine triphosphate to dihydroneopterin triphosphate, followed by the formation of 6-pyruvoyltetrahydropterin and its subsequent reduction to this compound.
Industrial Production Methods: Industrial production of this compound involves the fermentation of genetically modified microorganisms that overexpress the necessary enzymes. The fermentation broth is then subjected to purification processes to isolate and purify this compound .
Chemical Reactions Analysis
Types of Reactions: Tetrahydrobiopterin undergoes various chemical reactions, including oxidation, reduction, and substitution. It acts as a cofactor in hydroxylation reactions, where it facilitates the addition of hydroxyl groups to aromatic amino acids .
Common Reagents and Conditions: Common reagents used in reactions involving this compound include molecular oxygen and various hydroxylase enzymes. The reactions typically occur under physiological conditions, with the presence of specific enzymes being crucial for the reaction to proceed .
Major Products: The major products formed from reactions involving this compound include hydroxylated amino acids such as tyrosine and 5-hydroxytryptophan .
Scientific Research Applications
Comparison with Similar Compounds
Neopterin: A pteridine derivative involved in the immune response.
Dihydrobiopterin: An intermediate in the biosynthesis of tetrahydrobiopterin.
Sepiapterin: A precursor in the biosynthesis of this compound.
Uniqueness: this compound is unique in its ability to act as a cofactor for multiple hydroxylase enzymes, making it essential for the synthesis of several key neurotransmitters. Its role in both the degradation of phenylalanine and the biosynthesis of neurotransmitters distinguishes it from other similar compounds .
Properties
IUPAC Name |
2-amino-6-(1,2-dihydroxypropyl)-5,6,7,8-tetrahydro-3H-pteridin-4-one | |
---|---|---|
Source | PubChem | |
URL | https://pubchem.ncbi.nlm.nih.gov | |
Description | Data deposited in or computed by PubChem | |
InChI |
InChI=1S/C9H15N5O3/c1-3(15)6(16)4-2-11-7-5(12-4)8(17)14-9(10)13-7/h3-4,6,12,15-16H,2H2,1H3,(H4,10,11,13,14,17) | |
Source | PubChem | |
URL | https://pubchem.ncbi.nlm.nih.gov | |
Description | Data deposited in or computed by PubChem | |
InChI Key |
FNKQXYHWGSIFBK-UHFFFAOYSA-N | |
Source | PubChem | |
URL | https://pubchem.ncbi.nlm.nih.gov | |
Description | Data deposited in or computed by PubChem | |
Canonical SMILES |
CC(C(C1CNC2=C(N1)C(=O)NC(=N2)N)O)O | |
Source | PubChem | |
URL | https://pubchem.ncbi.nlm.nih.gov | |
Description | Data deposited in or computed by PubChem | |
Molecular Formula |
C9H15N5O3 | |
Source | PubChem | |
URL | https://pubchem.ncbi.nlm.nih.gov | |
Description | Data deposited in or computed by PubChem | |
DSSTOX Substance ID |
DTXSID80872469 | |
Record name | 5,6,7,8-Tetrahydrobiopterin | |
Source | EPA DSSTox | |
URL | https://comptox.epa.gov/dashboard/DTXSID80872469 | |
Description | DSSTox provides a high quality public chemistry resource for supporting improved predictive toxicology. | |
Molecular Weight |
241.25 g/mol | |
Source | PubChem | |
URL | https://pubchem.ncbi.nlm.nih.gov | |
Description | Data deposited in or computed by PubChem | |
CAS No. |
17528-72-2 | |
Record name | Tetrahydrobiopterin | |
Source | CAS Common Chemistry | |
URL | https://commonchemistry.cas.org/detail?cas_rn=17528-72-2 | |
Description | CAS Common Chemistry is an open community resource for accessing chemical information. Nearly 500,000 chemical substances from CAS REGISTRY cover areas of community interest, including common and frequently regulated chemicals, and those relevant to high school and undergraduate chemistry classes. This chemical information, curated by our expert scientists, is provided in alignment with our mission as a division of the American Chemical Society. | |
Explanation | The data from CAS Common Chemistry is provided under a CC-BY-NC 4.0 license, unless otherwise stated. | |
Record name | Tetrahydrobiopterin | |
Source | ChemIDplus | |
URL | https://pubchem.ncbi.nlm.nih.gov/substance/?source=chemidplus&sourceid=0017528722 | |
Description | ChemIDplus is a free, web search system that provides access to the structure and nomenclature authority files used for the identification of chemical substances cited in National Library of Medicine (NLM) databases, including the TOXNET system. | |
Record name | 5,6,7,8-Tetrahydrobiopterin | |
Source | EPA DSSTox | |
URL | https://comptox.epa.gov/dashboard/DTXSID80872469 | |
Description | DSSTox provides a high quality public chemistry resource for supporting improved predictive toxicology. | |
Synthesis routes and methods I
Procedure details
Synthesis routes and methods II
Procedure details
Synthesis routes and methods III
Procedure details
Synthesis routes and methods IV
Procedure details
Retrosynthesis Analysis
AI-Powered Synthesis Planning: Our tool employs the Template_relevance Pistachio, Template_relevance Bkms_metabolic, Template_relevance Pistachio_ringbreaker, Template_relevance Reaxys, Template_relevance Reaxys_biocatalysis model, leveraging a vast database of chemical reactions to predict feasible synthetic routes.
One-Step Synthesis Focus: Specifically designed for one-step synthesis, it provides concise and direct routes for your target compounds, streamlining the synthesis process.
Accurate Predictions: Utilizing the extensive PISTACHIO, BKMS_METABOLIC, PISTACHIO_RINGBREAKER, REAXYS, REAXYS_BIOCATALYSIS database, our tool offers high-accuracy predictions, reflecting the latest in chemical research and data.
Strategy Settings
Precursor scoring | Relevance Heuristic |
---|---|
Min. plausibility | 0.01 |
Model | Template_relevance |
Template Set | Pistachio/Bkms_metabolic/Pistachio_ringbreaker/Reaxys/Reaxys_biocatalysis |
Top-N result to add to graph | 6 |
Feasible Synthetic Routes
Disclaimer and Information on In-Vitro Research Products
Please be aware that all articles and product information presented on BenchChem are intended solely for informational purposes. The products available for purchase on BenchChem are specifically designed for in-vitro studies, which are conducted outside of living organisms. In-vitro studies, derived from the Latin term "in glass," involve experiments performed in controlled laboratory settings using cells or tissues. It is important to note that these products are not categorized as medicines or drugs, and they have not received approval from the FDA for the prevention, treatment, or cure of any medical condition, ailment, or disease. We must emphasize that any form of bodily introduction of these products into humans or animals is strictly prohibited by law. It is essential to adhere to these guidelines to ensure compliance with legal and ethical standards in research and experimentation.