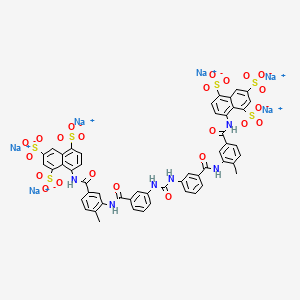
Suramin sodium
Overview
Description
Suramin sodium is a polysulfonated naphthylurea compound that was first developed by the German Bayer company in the 1910s. It is primarily used to treat African trypanosomiasis (sleeping sickness) and onchocerciasis (river blindness) caused by parasitic infections . This compound has also been explored for its potential in treating various viral infections and certain types of cancer .
Mechanism of Action
Target of Action
Suramin sodium, a polyanionic compound, has several primary targets. It acts as an antagonist of P2Y purinoceptor 2 and follicle-stimulating hormone receptors . It also inhibits NAD-dependent protein deacylase sirtuin-5, mitochondrial , and acts as an agonist of Ryanodine receptors . These targets play crucial roles in various cellular reactions, such as respiration and glycolysis .
Mode of Action
The exact mechanism of this compound is unknown, but its trypanocidal activity may be due to the inhibition of enzymes involved with the oxidation of reduced nicotinamide-adenine dinucleotide (NADH) . NADH functions as a co-enzyme in many cellular reactions, such as respiration and glycolysis, in the trypanosome parasite . Suramin’s action in the treatment of onchocerciasis is macrofilaricidal and partially microfilaricidal .
Biochemical Pathways
This compound impacts several biochemical pathways. It affects amino-acid, amino-sugar, and nucleotide-sugar metabolism . Time effects were observed on carbon intermediary metabolism, notably glycolysis and di- and tricarboxylic acids of the succinate production pathway and tricarboxylic acid (TCA) cycle .
Pharmacokinetics
This compound exhibits dose-proportional pharmacokinetics. After a single dose, the maximum plasma concentration (Cmax) and area under the plasma concentration-time curve from time zero to the time of the last measurable concentration (AUClast) increase in a dose-proportional manner . The plasma half-life (t1/2) is dose-independent, averaging 48 days (range 28-105 days) . This compound has a protein binding of 99.7% and a poor absorption . Its volume of distribution (Vd) is 90 ± 21 L (terminal phase); 38 ± 8 L (Steady state) .
Result of Action
This compound has demonstrated varied modes of action against Trypanosoma brucei . It has a dose- and time-dependent propensity to stimulate the adipogenic differentiation of rat preadipocytes isolated from the superficial fascia tissue and from adult adipose tissue . This stimulation is concomitant with a notable upregulation in expression levels of pivotal adipogenic factors as the adipocyte differentiation process unfolds .
Biochemical Analysis
Biochemical Properties
Suramin sodium plays a significant role in biochemical reactions by interacting with various enzymes, proteins, and other biomolecules. It inhibits enzymes involved in the oxidation of reduced nicotinamide-adenine dinucleotide (NADH), which is crucial for cellular respiration and glycolysis . Additionally, this compound acts as an antagonist of P2 receptors and an agonist of Ryanodine receptors, influencing cellular signaling pathways . It also inhibits follicle-stimulating hormone receptors and phospholipase A2, among others .
Cellular Effects
This compound affects various types of cells and cellular processes. It has been shown to stimulate adipocyte differentiation and promote adipogenesis in rat preadipocytes . This compound also exhibits antineoplastic effects by antagonizing various growth factors and cellular proteins . Furthermore, it can inhibit the replication of viruses by interfering with viral attachment, entry, and release from host cells .
Molecular Mechanism
The molecular mechanism of this compound involves several binding interactions and enzyme inhibitions. It inhibits enzymes involved in the oxidation of reduced NADH, which is essential for cellular respiration and glycolysis . This compound also acts as an antagonist of P2 receptors and an agonist of Ryanodine receptors, influencing calcium signaling pathways . Additionally, it inhibits follicle-stimulating hormone receptors and phospholipase A2, affecting various cellular functions .
Temporal Effects in Laboratory Settings
In laboratory settings, the effects of this compound change over time. It has a long half-life of approximately 48 days, with detectable levels in urine for more than 140 days following infusion . This compound demonstrates a dose- and time-dependent propensity to stimulate adipogenic differentiation of rat preadipocytes
Dosage Effects in Animal Models
The effects of this compound vary with different dosages in animal models. In a study on polycystic kidney disease in mice, a dosage of 60 mg/kg twice weekly significantly reduced renal cyst densities, cell proliferation, and macrophage infiltration . High doses of this compound can lead to nephrotoxicity and other adverse effects, indicating the need for careful dosage management .
Metabolic Pathways
This compound is involved in various metabolic pathways, including amino-acid, amino-sugar, and nucleotide-sugar metabolism . It affects carbon intermediary metabolism, notably glycolysis and the tricarboxylic acid (TCA) cycle . This compound’s impact on these pathways highlights its broad metabolic influence and potential therapeutic applications.
Transport and Distribution
This compound is poorly absorbed from the gastrointestinal tract and is widely distributed to tissues, including the kidneys . It has a volume of distribution of 31–46 liters and is primarily excreted via the kidneys . The drug’s long half-life and extensive tissue distribution contribute to its prolonged therapeutic effects and potential toxicity.
Subcellular Localization
The subcellular localization of this compound involves its distribution within various cellular compartments. It can target specific cellular structures, such as the mitochondria and endoplasmic reticulum, influencing their function and activity
Preparation Methods
The preparation of suramin sodium involves several synthetic routes and reaction conditions. One method includes the acylation of 1-amido-4,6,8-naphthalene trisulfonic acid trisodium with 4-methyl-3-nitrobenzoyl chloride, followed by reduction using palladium on carbon (Pd/C) catalytic hydrogenation . This method replaces the traditional iron powder reduction and uses 1,1’-carbonyldiimidazole instead of highly toxic phosgene, making the process safer and more suitable for industrial production .
Chemical Reactions Analysis
Suramin sodium undergoes various chemical reactions, including:
Reduction: The compound can be reduced using catalytic hydrogenation, as mentioned in its preparation methods.
Substitution: This compound can participate in substitution reactions, particularly involving its sulfonic acid groups.
Common reagents and conditions used in these reactions include palladium on carbon for hydrogenation and various sulfonating agents for substitution reactions. The major products formed depend on the specific reaction conditions and reagents used.
Scientific Research Applications
Suramin sodium has a wide range of scientific research applications:
Comparison with Similar Compounds
Suramin sodium is unique due to its polysulfonated naphthylurea structure and broad spectrum of enzymatic actions. Similar compounds include:
Heparin: Like this compound, heparin is a polyanionic compound with similar adipogenic effects.
PPADS, NF023, and NF449: These are suramin analogs containing different numbers of sulfonic acid groups, which influence their biological activities.
This compound stands out for its versatility in treating parasitic infections, viral diseases, and its potential in cancer therapy .
Properties
Key on ui mechanism of action |
The mechanism is unknown, but the trypanocidal activity may be due to the inhibition of enzymes involved with the oxidation of reduced nicotinamide-adenine dinucleotide (NADH), which functions as a co-enzyme in many cellular reactions, such as respiration and glycolysis, in the trypanosome parasite. Suramin's action in the treatment of onchocerciasis is macrofilaricidal and partially microfilaricidal. It may also act as an antagonist of P2 receptors and as an agonist of Ryanodine receptors. It also can inhibit follicle-stimulating hormone receptors. |
---|---|
CAS No. |
129-46-4 |
Molecular Formula |
C51H40N6NaO23S6 |
Molecular Weight |
1320.3 g/mol |
IUPAC Name |
8-[[4-methyl-3-[[3-[[3-[[2-methyl-5-[(4,6,8-trisulfonaphthalen-1-yl)carbamoyl]phenyl]carbamoyl]phenyl]carbamoylamino]benzoyl]amino]benzoyl]amino]naphthalene-1,3,5-trisulfonic acid |
InChI |
InChI=1S/C51H40N6O23S6.Na/c1-25-9-11-29(49(60)54-37-13-15-41(83(69,70)71)35-21-33(81(63,64)65)23-43(45(35)37)85(75,76)77)19-39(25)56-47(58)27-5-3-7-31(17-27)52-51(62)53-32-8-4-6-28(18-32)48(59)57-40-20-30(12-10-26(40)2)50(61)55-38-14-16-42(84(72,73)74)36-22-34(82(66,67)68)24-44(46(36)38)86(78,79)80;/h3-24H,1-2H3,(H,54,60)(H,55,61)(H,56,58)(H,57,59)(H2,52,53,62)(H,63,64,65)(H,66,67,68)(H,69,70,71)(H,72,73,74)(H,75,76,77)(H,78,79,80); |
InChI Key |
HWRBYSXGEWIANS-UHFFFAOYSA-N |
SMILES |
CC1=C(C=C(C=C1)C(=O)NC2=C3C(=CC(=CC3=C(C=C2)S(=O)(=O)[O-])S(=O)(=O)[O-])S(=O)(=O)[O-])NC(=O)C4=CC(=CC=C4)NC(=O)NC5=CC=CC(=C5)C(=O)NC6=C(C=CC(=C6)C(=O)NC7=C8C(=CC(=CC8=C(C=C7)S(=O)(=O)[O-])S(=O)(=O)[O-])S(=O)(=O)[O-])C.[Na+].[Na+].[Na+].[Na+].[Na+].[Na+] |
Canonical SMILES |
CC1=C(C=C(C=C1)C(=O)NC2=C3C(=CC(=CC3=C(C=C2)S(=O)(=O)O)S(=O)(=O)O)S(=O)(=O)O)NC(=O)C4=CC(=CC=C4)NC(=O)NC5=CC=CC(=C5)C(=O)NC6=C(C=CC(=C6)C(=O)NC7=C8C(=CC(=CC8=C(C=C7)S(=O)(=O)O)S(=O)(=O)O)S(=O)(=O)O)C.[Na] |
Appearance |
Solid powder |
Key on ui other cas no. |
129-46-4 |
physical_description |
Solid |
Pictograms |
Irritant |
Purity |
>95% (or refer to the Certificate of Analysis) |
Related CAS |
129-46-4 (hexa-hydrochloride salt) |
shelf_life |
>2 years if stored properly |
solubility |
8.72e-03 g/L |
storage |
Dry, dark and at 0 - 4 C for short term (days to weeks) or -20 C for long term (months to years). |
Synonyms |
Germanin Hexasodium Salt Suramin Monosodium Salt Suramin Moranil Naganin Naganol Naphuride Salt Suramin, Hexasodium Salt Suramin, Monosodium Sodium, Suramin Suramin Suramin Sodium Suramin, Hexasodium Salt Suramin, Monosodium Salt |
Origin of Product |
United States |
Retrosynthesis Analysis
AI-Powered Synthesis Planning: Our tool employs the Template_relevance Pistachio, Template_relevance Bkms_metabolic, Template_relevance Pistachio_ringbreaker, Template_relevance Reaxys, Template_relevance Reaxys_biocatalysis model, leveraging a vast database of chemical reactions to predict feasible synthetic routes.
One-Step Synthesis Focus: Specifically designed for one-step synthesis, it provides concise and direct routes for your target compounds, streamlining the synthesis process.
Accurate Predictions: Utilizing the extensive PISTACHIO, BKMS_METABOLIC, PISTACHIO_RINGBREAKER, REAXYS, REAXYS_BIOCATALYSIS database, our tool offers high-accuracy predictions, reflecting the latest in chemical research and data.
Strategy Settings
Precursor scoring | Relevance Heuristic |
---|---|
Min. plausibility | 0.01 |
Model | Template_relevance |
Template Set | Pistachio/Bkms_metabolic/Pistachio_ringbreaker/Reaxys/Reaxys_biocatalysis |
Top-N result to add to graph | 6 |
Feasible Synthetic Routes
Q1: What is the primary mechanism of action of suramin sodium?
A1: this compound exhibits its activity through multiple mechanisms. It disrupts the binding of various growth factors, including insulin-like growth factor I (IGF-I), epidermal growth factor (EGF), platelet-derived growth factor (PDGF), and tumor growth factor-beta (TGF-beta) to their receptors []. This inhibition of growth factor signaling can hinder cell proliferation and migration, potentially impacting tumor growth and angiogenesis. Additionally, this compound inhibits retroviral reverse transcriptase, uncouples G-proteins from receptors, and affects topoisomerases, cellular folate transport, and steroidogenesis [].
Q2: How does this compound affect cellular folate transport?
A2: this compound competitively inhibits the influx of methotrexate, an antifolate drug, through the reduced-folate carrier system in tumor cells []. This inhibition has been shown to be highly specific to this compound compared to other arylsulfonic acids, indicating a particular interaction with the reduced-folate carrier [].
Q3: What is the molecular formula and weight of this compound?
A3: The molecular formula of this compound is C51H34N6Na6O23S6, and its molecular weight is 1429.16 g/mol [].
Q4: Does the available research discuss any specific catalytic properties of this compound?
A4: The provided research articles do not delve into the catalytic properties of this compound. The focus is primarily on its biological activities and applications as an antiparasitic, antiviral, and potential antineoplastic agent.
Q5: Has computational chemistry been utilized to study this compound?
A5: While the provided articles don't directly employ computational chemistry for this compound, one study utilizes docking simulations to investigate the binding interactions of flavanol-fatty alcohol hybrids (structurally unrelated to suramin) with α-glucosidase and PTP1B enzymes []. This approach could potentially be applied to this compound in future research to understand its interactions with target proteins.
Q6: Does modifying the structure of this compound affect its activity?
A6: The provided articles do not specifically address the SAR of this compound. Further research is needed to understand how modifications to its structure might influence its activity, potency, and selectivity.
Q7: Are there any formulation strategies mentioned for improving the stability or bioavailability of this compound?
A7: The provided articles primarily focus on the direct administration of this compound and don't delve into specific formulation strategies to enhance its stability or bioavailability. This area presents an opportunity for future research to optimize its therapeutic application.
Q8: What information is available regarding SHE regulations for this compound?
A8: The provided articles primarily focus on the scientific and medical aspects of this compound and do not provide details regarding specific SHE regulations. It is crucial to consult relevant regulatory guidelines and agencies for comprehensive information on handling, storage, and disposal of this compound.
Q9: What is the pharmacokinetic profile of this compound?
A9: Following a single intravenous dose in healthy Chinese volunteers, this compound exhibited dose-proportional increases in Cmax and AUClast []. The plasma half-life (t1/2) remained independent of the dose, averaging 48 days with a range of 28–105 days []. Urinary excretion of this compound was low, with less than 4% of the dose recovered in urine within 7 days post-administration []. Notably, this compound was detectable in urine samples for extended periods, exceeding 140 days after infusion [].
Q10: What are the in vitro and in vivo efficacies of this compound against Trypanosoma evansi?
A10: In a study involving four Himalayan black bears infected with Trypanosoma evansi, treatment with this compound at twice the recommended dosage for domestic animals (10 mg/kg) administered twice at 3-day intervals successfully cleared the parasite from the bloodstream []. All four bears tested aparasitemic on days 5, 7, and 10 post-treatment, demonstrating the in vivo efficacy of this compound against T. evansi in this animal model [].
Q11: Has this compound shown any promising results in cancer treatment?
A11: While this compound hasn't shown significant antitumor activity as a single agent in metastatic renal cell carcinoma [], there have been observations suggesting potential benefits. In one patient, autopsy revealed over 90% necrosis of tumor sites following this compound treatment []. Additionally, another patient experienced normalization of tumor-related hypercalcemia []. These findings, though limited, warrant further investigation into this compound's potential role in cancer therapy, perhaps in combination with other agents.
Q12: Are there known resistance mechanisms associated with this compound?
A12: Yes, resistance to this compound is primarily attributed to alterations in the parasite's transport proteins, specifically those involved in drug uptake []. This mechanism hinders the drug's ability to reach its intracellular targets and exert its effects.
Q13: What are the common side effects associated with this compound therapy?
A13: this compound treatment can lead to various side effects, with the most frequent being fever, rash, malaise, nausea, neurological symptoms, and vomiting []. Additionally, it can cause hematological toxicities such as neutropenia and thrombocytopenia, as well as liver dysfunction, renal insufficiency, adrenal insufficiency, and ocular complications like vortex keratopathy [, , ]. The severity of these side effects can vary and necessitates careful monitoring during treatment.
Q14: Are there any specific drug delivery systems designed for this compound?
A14: Yes, one study explores the use of a bone-targeted and pH-sensitive drug delivery system for this compound []. This system utilizes alendronate-conjugated polymeric nanoparticles to encapsulate and deliver this compound specifically to bone lesions, aiming to enhance its therapeutic efficacy in treating fibrous dysplasia [].
Disclaimer and Information on In-Vitro Research Products
Please be aware that all articles and product information presented on BenchChem are intended solely for informational purposes. The products available for purchase on BenchChem are specifically designed for in-vitro studies, which are conducted outside of living organisms. In-vitro studies, derived from the Latin term "in glass," involve experiments performed in controlled laboratory settings using cells or tissues. It is important to note that these products are not categorized as medicines or drugs, and they have not received approval from the FDA for the prevention, treatment, or cure of any medical condition, ailment, or disease. We must emphasize that any form of bodily introduction of these products into humans or animals is strictly prohibited by law. It is essential to adhere to these guidelines to ensure compliance with legal and ethical standards in research and experimentation.