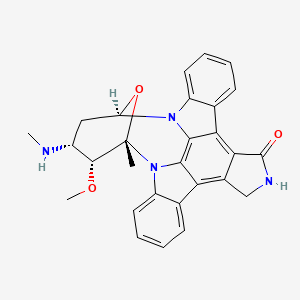
Staurosporine
Overview
Description
Staurosporine is a broad-spectrum kinase inhibitor originally isolated from Streptomyces species. It exhibits potent activity against multiple protein kinases, including protein kinase C (PKC), tyrosine kinases, and cyclin-dependent kinases (CDKs), with IC50 values typically in the low nanomolar range . Its planar indolocarbazole structure enables tight binding to the ATP pocket of kinases, making it a valuable tool for studying kinase signaling pathways . Despite its strong inhibitory activity, this compound’s lack of selectivity limits its clinical use, prompting the development of analogs with improved specificity .
Preparation Methods
Synthetic Routes and Reaction Conditions: Staurosporine can be synthesized through a series of complex organic reactions. One of the key steps involves the formation of the indolocarbazole core, which is achieved through a sequence of C-H functionalization reactions . The synthetic route typically involves the use of copper-catalyzed arylations and other selective functionalization reactions to build the intricate structure of this compound .
Industrial Production Methods: Industrial production of this compound primarily relies on fermentation processes using genetically engineered strains of Streptomyces species . High-titer production has been achieved through the integration of multi-copy biosynthetic gene clusters into heterologous hosts like Streptomyces albus . Optimization of fermentation conditions, such as pH control and glucose feeding, has led to significant improvements in yield .
Chemical Reactions Analysis
Types of Reactions: Staurosporine undergoes various chemical reactions, including oxidation, reduction, and substitution . It is particularly known for its ability to inhibit protein kinases by preventing ATP binding .
Common Reagents and Conditions: Common reagents used in the synthesis and modification of this compound include copper catalysts for arylation reactions and various oxidizing and reducing agents . The reaction conditions often involve controlled temperatures and pH levels to ensure the stability of the compound .
Major Products: The major products formed from the reactions involving this compound include various derivatives that retain the indolocarbazole core structure . These derivatives are often studied for their enhanced biological activities and potential therapeutic applications .
Scientific Research Applications
Cancer Research Applications
Staurosporine has been extensively studied for its anticancer properties. Several case studies highlight its efficacy against various cancer types:
Case Study: Oral Carcinoma
In a study involving human papillomavirus-positive oral carcinoma cells (KB), this compound demonstrated an IC50 value of approximately 100 nM, effectively inducing apoptosis and disrupting mitochondrial membrane potential .
Case Study: Breast Cancer
Research indicates that this compound reduces the viability of HER2-positive breast cancer cells, showing synergistic effects when combined with other inhibitors like lapatinib . This suggests its potential role in overcoming resistance to standard therapies.
Table 1: Summary of this compound's Anticancer Effects
Cancer Type | Cell Line | IC50 (nM) | Mechanism of Action |
---|---|---|---|
Oral Carcinoma | KB | 100 | Induces apoptosis via mitochondrial pathways |
Breast Cancer | HER2-positive | Varies | Reduces cell viability; synergistic with lapatinib |
Leukemia | U-937 | 0.5-1 | Induces G2/M arrest and activates caspase-3 |
Neuroprotective Applications
This compound has also been investigated for its neuroprotective properties. In a pharmacological screening approach, it was identified as a compound that can protect neurons from ischemic injury by modulating apoptotic pathways .
Case Study: Dopaminergic Neurite Outgrowth
A study reported that this compound induces neurite outgrowth in dopaminergic neurons, indicating its potential use in neurodegenerative disease models . This effect suggests that this compound may help enhance neuronal recovery post-injury.
Development of this compound Derivatives
The quest for more selective compounds has led to the development of this compound derivatives aimed at improving therapeutic efficacy while minimizing side effects. For instance, derivatives have been shown to exhibit enhanced activity against prostate cancer cells while selectively inhibiting specific kinases such as PKC theta and ROCK2 .
Table 2: this compound Derivatives and Their Applications
Derivative Name | Target Disease | Key Activity |
---|---|---|
This compound Derivative A | Prostate Cancer | Higher activity on prostate cancer cells |
This compound Derivative B | Colon Cancer | Inhibits PKC theta and ROCK2 |
Broader Implications in Pharmacology
This compound's ability to inhibit a wide range of kinases makes it a valuable tool for probing cellular signaling networks. Its application extends beyond oncology into areas such as:
Mechanism of Action
Staurosporine exerts its effects primarily through the inhibition of protein kinases by preventing ATP binding to the kinase . This inhibition disrupts various cellular signaling pathways, leading to effects such as apoptosis . The compound has a high affinity for many kinases but lacks selectivity, which limits its clinical use . One of the key pathways involved in its mechanism of action is the activation of caspase-3, which plays a crucial role in the induction of apoptosis .
Comparison with Similar Compounds
Structural and Functional Comparison with Similar Compounds
Staurosporine shares structural homology with several microbial and synthetic analogs, which differ in substituents and stereochemistry, leading to variations in kinase selectivity and biological effects. Key compounds are compared below:
Table 1: Structural and Functional Comparison of this compound and Analogs
Key Research Findings
Kinase Selectivity and Mechanisms
- This compound vs. K-252a : While both inhibit PKC, K-252a shows higher selectivity for TrkA and phosphorylase kinase. Structural differences (methyl ester vs. methoxy group) reduce K-252a’s ability to induce neuronal differentiation in RGC-5 cells .
- This compound vs. UCN-01 : The 7-hydroxyl group in UCN-01 enhances selectivity for checkpoint kinase 1 (Chk1), making it a promising anti-cancer agent. Both compounds induce neuronal differentiation via their 3’-methoxy and adjacent amine groups .
- This compound vs. PKC-412 : PKC-412’s benzoyl group sterically hinders interactions critical for differentiation, despite retaining PKC inhibition .
Critical Analysis of Selectivity and Limitations
This compound’s pan-kinase activity arises from its ability to form hydrogen bonds with conserved kinase hinge regions (e.g., Glu239 and Leu241 in DYRK1A) . Analogs like UCN-01 and PKC-412 mitigate this promiscuity through targeted modifications but face trade-offs in potency or off-target effects. For example:
- K-252a ’s partial differentiation activity underscores the necessity of the 3’-methoxy-amine motif for full neuronal signaling .
Biological Activity
Staurosporine is a potent and versatile compound originally isolated from the bacterium Streptomyces staurosporeus. It has garnered significant attention in the field of pharmacology due to its diverse biological activities, particularly its role as a protein kinase inhibitor and its effects on cell proliferation and apoptosis. This article provides an in-depth examination of the biological activity of this compound, including its mechanisms of action, therapeutic potential, and relevant case studies.
This compound acts primarily as a non-selective inhibitor of protein kinases, particularly targeting protein kinase C (PKC) and cyclin-dependent kinases (CDKs). Its ability to inhibit these kinases leads to various cellular responses, including:
- Induction of Apoptosis : this compound triggers intrinsic apoptotic pathways in various cancer cell lines. For instance, in U-937 cells, treatment with this compound resulted in significant activation of caspase-3, a key enzyme involved in the apoptosis process. The cleavage of procaspase-3 correlates with increased cell death, indicating that this compound effectively induces apoptosis through caspase activation .
- Cell Cycle Arrest : this compound has been shown to cause G2/M phase arrest in cancer cells. This effect is associated with the downregulation of cyclins and CDKs, which are crucial for cell cycle progression . Table 1 summarizes the effects of this compound on cell cycle regulation.
Cell Line | Dose (μM) | Duration (hours) | Cell Cycle Phase Arrested | Key Findings |
---|---|---|---|---|
U-937 | 0.5 | 18 | G1 | Induction of apoptosis via caspase-3 activation |
U-937 | 1 | 24 | G2/M | Increased cell death and downregulation of cyclins |
Various | Variable | Variable | G2/M | Non-selective inhibition of PKC and CDKs |
Neuroprotective Effects
Research indicates that this compound may also possess neuroprotective properties. In studies involving primary cortical neurons subjected to oxygen-glucose deprivation, this compound demonstrated a reduction in caspase-3 activity, suggesting a protective effect against neuronal apoptosis . This highlights its potential therapeutic applications in neurodegenerative diseases.
Antitumor Activity
This compound has been extensively studied for its antitumor properties. It inhibits tumor cell growth across various cancer types through multiple mechanisms:
- Inhibition of Tumor Growth : this compound has shown significant antiproliferative activity against several human cancer cell lines. Its mechanism includes the induction of apoptosis and modulation of survival pathways critical for cancer cell resistance .
- Development of Analogues : Research into this compound derivatives has indicated that modifications at specific positions can enhance selectivity and potency against particular tumor cells. For example, halogenation at the 3-position has been associated with increased antitumor activity .
Case Studies
Several case studies have highlighted the efficacy of this compound in clinical settings:
- Leukemia Treatment : A study involving U-937 leukemia cells demonstrated that this compound effectively induces apoptosis while inhibiting proliferation pathways. The findings suggest that this compound could be a valuable candidate for treating hematological malignancies .
- Neuroprotection in Ischemia : In models of ischemic stroke, this compound exhibited neuroprotective effects when administered post-injury, indicating its potential for therapeutic use in acute neurological conditions .
Q & A
Basic Research Questions
Q. How is staurosporine used to induce apoptosis in cell culture studies?
this compound is commonly applied at concentrations ranging from 100–500 nM to trigger apoptosis. Cell viability is typically assessed using assays like MTT (3-(4,5-dimethylthiazol-2-yl)-2,5-diphenyltetrazolium bromide) or lactate dehydrogenase (LDH) release to quantify cytotoxicity. For example, in PC12 cells, 500 nM this compound reduced viability by ~60% within 24 hours, validated via dose-response curves . Controls such as vehicle (e.g., DMSO) and untreated samples are critical to isolate compound-specific effects .
Q. What are the standard protocols for assessing this compound’s kinase inhibition?
Kinase inhibition is evaluated using in vitro kinase assays with purified enzymes (e.g., PKC, PKA) and ATP-competitive substrates. Activity is quantified via radioactivity-based methods (e.g., γ-³²P-ATP incorporation) or fluorescence polarization. This compound’s IC₅₀ values vary widely (nM to µM) depending on kinase isoform, necessitating parallel controls with selective inhibitors (e.g., herbimycin for Src kinases) .
Q. How should researchers determine the optimal this compound concentration for a new cell line?
Conduct dose-response experiments using a logarithmic concentration range (e.g., 1 nM–1 µM). Calculate IC₅₀ values using nonlinear regression analysis (e.g., GraphPad Prism). For apoptosis studies, validate with caspase-3/7 activation assays or Annexin V staining to confirm mechanistic specificity .
Advanced Research Questions
Q. How can this compound’s off-target kinase cross-reactivity be mitigated in experimental design?
Combine this compound with isoform-specific kinase inhibitors (e.g., gefitinib for EGFR) or use genetic approaches (e.g., siRNA knockdown) to isolate target pathways. Phospho-specific antibodies (e.g., anti-ASK1 [phospho T838]) can validate downstream signaling changes via flow cytometry or Western blot . Additionally, computational modeling (e.g., parameter fitting for Bcl-2 family proteins) helps distinguish direct from indirect effects .
Q. What strategies address contradictions in this compound’s reported IC₅₀ values across studies?
Variability arises from differences in kinase sources (recombinant vs. endogenous), ATP concentrations, and assay conditions. Standardize protocols using commercially validated kinase kits (e.g., Eurofins KinaseProfiler) and report ATP levels (e.g., Km-adjusted assays). Cross-validate findings with orthogonal methods, such as thermal shift assays or cellular kinome profiling .
Q. How can this compound be integrated into combination therapy models for cancer research?
Pre-treat cells with pathway-specific inhibitors (e.g., Wnt-3a peptide) to modulate this compound’s effects. For example, Wnt-3a (100 ng/mL) reduced this compound-induced cytotoxicity by 30% in PC12 cells, suggesting synergistic/antagonistic interactions . Use kinetic modeling (e.g., ordinary differential equations) to predict drug interactions and optimize dosing schedules .
Q. What methods ensure reproducibility in this compound batch-to-batch variability?
Source this compound from certified suppliers (e.g., Sigma-Aldrich, Tocris) and document lot numbers. Verify activity via kinase inhibition assays (e.g., PKCα IC₅₀ ≈ 2–5 nM) and characterize purity via HPLC. Store aliquots at −80°C in anhydrous DMSO to prevent degradation .
Q. Methodological Notes
- Data Analysis : Use ANOVA with post-hoc tests (e.g., Newman-Keuls) for multi-group comparisons in viability assays .
- Ethical Reporting : Adhere to journal guidelines (e.g., Beilstein Journal) for experimental replication, including detailed supplementary methods .
- Contradiction Resolution : Systematically review kinase assay conditions and cellular contexts when reconciling conflicting data .
Properties
CAS No. |
62996-74-1 |
---|---|
Molecular Formula |
C28H26N4O3 |
Molecular Weight |
466.5 g/mol |
IUPAC Name |
(2S)-3-methoxy-2-methyl-4-(methylamino)-29-oxa-1,7,17-triazaoctacyclo[12.12.2.12,6.07,28.08,13.015,19.020,27.021,26]nonacosa-8,10,12,14,19,21,23,25,27-nonaen-16-one |
InChI |
InChI=1S/C28H26N4O3/c1-28-26(34-3)17(29-2)12-20(35-28)31-18-10-6-4-8-14(18)22-23-16(13-30-27(23)33)21-15-9-5-7-11-19(15)32(28)25(21)24(22)31/h4-11,17,20,26,29H,12-13H2,1-3H3,(H,30,33)/t17?,20?,26?,28-/m0/s1 |
InChI Key |
HKSZLNNOFSGOKW-ZYSRIHRCSA-N |
SMILES |
CC12C(C(CC(O1)N3C4=CC=CC=C4C5=C6C(=C7C8=CC=CC=C8N2C7=C53)CNC6=O)NC)OC |
Isomeric SMILES |
C[C@@]12C(C(CC(O1)N3C4=CC=CC=C4C5=C6C(=C7C8=CC=CC=C8N2C7=C53)CNC6=O)NC)OC |
Canonical SMILES |
CC12C(C(CC(O1)N3C4=CC=CC=C4C5=C6C(=C7C8=CC=CC=C8N2C7=C53)CNC6=O)NC)OC |
Appearance |
Solid powder |
Key on ui other cas no. |
62996-74-1 |
Pictograms |
Flammable; Acute Toxic; Irritant; Health Hazard; Environmental Hazard |
Purity |
>98% (or refer to the Certificate of Analysis) |
shelf_life |
>2 years if stored properly |
solubility |
Soluble in DMSO, not in water |
storage |
Dry, dark and at 0 - 4 C for short term (days to weeks) or -20 C for long term (months to years). |
Synonyms |
8,12-Epoxy-1H,8H-2,7b,12a-triazadibenzo(a,g)cyclonona(cde)trinden-1-one, 2,3,9,10,11,12-hexahydro-9-methoxy-8-methyl-10-(methylamino)-, (8alpha,9beta,10beta,12alpha)-(+)- Staurosporine |
Origin of Product |
United States |
Retrosynthesis Analysis
AI-Powered Synthesis Planning: Our tool employs the Template_relevance Pistachio, Template_relevance Bkms_metabolic, Template_relevance Pistachio_ringbreaker, Template_relevance Reaxys, Template_relevance Reaxys_biocatalysis model, leveraging a vast database of chemical reactions to predict feasible synthetic routes.
One-Step Synthesis Focus: Specifically designed for one-step synthesis, it provides concise and direct routes for your target compounds, streamlining the synthesis process.
Accurate Predictions: Utilizing the extensive PISTACHIO, BKMS_METABOLIC, PISTACHIO_RINGBREAKER, REAXYS, REAXYS_BIOCATALYSIS database, our tool offers high-accuracy predictions, reflecting the latest in chemical research and data.
Strategy Settings
Precursor scoring | Relevance Heuristic |
---|---|
Min. plausibility | 0.01 |
Model | Template_relevance |
Template Set | Pistachio/Bkms_metabolic/Pistachio_ringbreaker/Reaxys/Reaxys_biocatalysis |
Top-N result to add to graph | 6 |
Feasible Synthetic Routes
Disclaimer and Information on In-Vitro Research Products
Please be aware that all articles and product information presented on BenchChem are intended solely for informational purposes. The products available for purchase on BenchChem are specifically designed for in-vitro studies, which are conducted outside of living organisms. In-vitro studies, derived from the Latin term "in glass," involve experiments performed in controlled laboratory settings using cells or tissues. It is important to note that these products are not categorized as medicines or drugs, and they have not received approval from the FDA for the prevention, treatment, or cure of any medical condition, ailment, or disease. We must emphasize that any form of bodily introduction of these products into humans or animals is strictly prohibited by law. It is essential to adhere to these guidelines to ensure compliance with legal and ethical standards in research and experimentation.