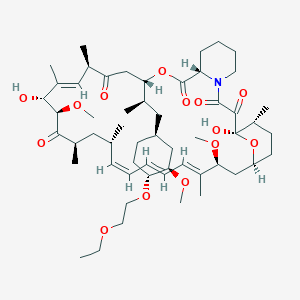
Umirolimus
Overview
Description
Umirolimus, also known as Biolimus, is an immunosuppressant, a macrocyclic lactone, and a highly lipophilic derivative of sirolimus . It is proprietary to Biosensors International, which uses it in its own drug-eluting stents . Umirolimus inhibits T cell and smooth muscle cell proliferation .
Molecular Structure Analysis
Umirolimus has a molecular formula of C55H87NO14 and an average mass of 986.278 Da . It has 15 defined stereocenters .Physical And Chemical Properties Analysis
Umirolimus has a molecular formula of C55H87NO14, an average mass of 986.278 Da, and a mono-isotopic mass of 985.612671 Da . It has 15 defined stereocenters .Scientific Research Applications
Immunosuppressant
Umirolimus is an immunosuppressant, a macrocyclic lactone, and a highly lipophilic derivative of sirolimus . It inhibits T cell and smooth muscle cell proliferation .
Drug-Eluting Stents
Umirolimus is used in drug-eluting stents. It is proprietary to Biosensors International, which uses it in its own drug-eluting stents, and licenses it to partners such as Terumo . The drug is more rapidly absorbed by the vessel wall, readily attaches and enters smooth muscle cell membranes causing cell cycle arrest at G0, and is comparable to sirolimus in terms of potency .
Treatment of Coronary Artery Aneurysms
A case report showed that a 51-year-old man developed coronary artery aneurysms (CAAs) following placement of a umirolimus-eluting stent . This suggests that umirolimus could potentially be used in the treatment of coronary artery aneurysms.
Vascular Stent Technologies
Biolimus A9™ (BA9™) was designed specifically for vascular stent technologies . The Research and Development team developed several versions of Biolimus, through a number of iterations ultimately decided to focus on BA9™ (Biolimus A9™), as the best option for coronary stent application .
Treatment of In-Stent Restenosis
A novel drug-coated balloon (DCB) coated with Biolimus A9 represents an alternative to traditional paclitaxel- and sirolimus-coated devices . It has been investigated for the safety and efficacy of this novel DCB in the treatment of coronary in-stent restenosis .
Enhanced Local Drug Delivery
Biolimus A9™ (BA9™) is more lipophilic due to regioselective modification for more targeted effect . It has greater local bioavailability than Sirolimus and Everolimus . Its structure slows drug metabolism by changing the affinity for enzymatic elimination .
Mechanism of Action
Target of Action
Umirolimus, also known as Biolimus A9, primarily targets the mammalian target of rapamycin (mTOR) . mTOR is a serine/threonine-protein kinase that plays a crucial role in regulating cell growth, proliferation, and survival .
Mode of Action
Umirolimus is an immunosuppressant and a highly lipophilic derivative of sirolimus . It inhibits T cell and smooth muscle cell proliferation . This analog has a chemical modification at position 40 of the rapamycin ring . It has potent immunosuppressive properties that are similar to those of sirolimus, but the drug is more rapidly absorbed by the vessel wall, readily attaches, and enters smooth muscle cell membranes causing cell cycle arrest at G0 .
Biochemical Pathways
The key biological event associated with the restenotic process is the proliferation of smooth muscle cells in response to the expansion of a foreign body against the vessel wall . This proliferative response is initiated by the early expression of growth factors such as PDGF isoforms, bFGF, thrombin, which bind to cellular receptors . The signal transduction events which culminate in cell cycle arrest in the G1 phase are initiated as a result of ligand binding to an immunophilin known as FK binding protein-12 .
Pharmacokinetics
It is known that umirolimus is a highly lipophilic compound , which suggests that it may have good absorption and distribution characteristics. More research is needed to fully understand the ADME properties of Umirolimus.
Result of Action
The primary molecular effect of Umirolimus is the inhibition of T cell and smooth muscle cell proliferation . This leads to cell cycle arrest at G0 . On a cellular level, this results in the prevention of restenosis, a condition where an artery that has been opened by angioplasty closes again .
Future Directions
While specific future directions for Umirolimus are not detailed in the search results, it’s known that new generations of drug-eluting stents (DES) are being designed to overcome the limitations of first-generation DES, which includes Umirolimus .
Relevant Papers One case report discusses a 51-year-old man who developed coronary artery aneurysms following the placement of a Umirolimus-eluting stent . Another source mentions Umirolimus as a research topic with over seven publications .
properties
IUPAC Name |
(1R,9S,12S,15R,16E,18R,19R,21R,23S,24E,26E,28E,30S,32S,35R)-12-[(2R)-1-[(1S,3R,4R)-4-(2-ethoxyethoxy)-3-methoxycyclohexyl]propan-2-yl]-1,18-dihydroxy-19,30-dimethoxy-15,17,21,23,29,35-hexamethyl-11,36-dioxa-4-azatricyclo[30.3.1.04,9]hexatriaconta-16,24,26,28-tetraene-2,3,10,14,20-pentone | |
---|---|---|
Source | PubChem | |
URL | https://pubchem.ncbi.nlm.nih.gov | |
Description | Data deposited in or computed by PubChem | |
InChI |
InChI=1S/C55H87NO14/c1-12-67-26-27-68-45-24-22-41(31-48(45)65-10)30-37(5)47-33-44(57)36(4)29-39(7)50(59)51(66-11)49(58)38(6)28-34(2)18-14-13-15-19-35(3)46(64-9)32-42-23-21-40(8)55(63,70-42)52(60)53(61)56-25-17-16-20-43(56)54(62)69-47/h13-15,18-19,29,34,36-38,40-43,45-48,50-51,59,63H,12,16-17,20-28,30-33H2,1-11H3/b15-13+,18-14+,35-19+,39-29+/t34-,36-,37-,38-,40-,41+,42+,43+,45-,46+,47+,48-,50-,51+,55-/m1/s1 | |
Source | PubChem | |
URL | https://pubchem.ncbi.nlm.nih.gov | |
Description | Data deposited in or computed by PubChem | |
InChI Key |
YYSFXUWWPNHNAZ-PKJQJFMNSA-N | |
Source | PubChem | |
URL | https://pubchem.ncbi.nlm.nih.gov | |
Description | Data deposited in or computed by PubChem | |
Canonical SMILES |
CCOCCOC1CCC(CC1OC)CC(C)C2CC(=O)C(C=C(C(C(C(=O)C(CC(C=CC=CC=C(C(CC3CCC(C(O3)(C(=O)C(=O)N4CCCCC4C(=O)O2)O)C)OC)C)C)C)OC)O)C)C | |
Source | PubChem | |
URL | https://pubchem.ncbi.nlm.nih.gov | |
Description | Data deposited in or computed by PubChem | |
Isomeric SMILES |
CCOCCO[C@@H]1CC[C@H](C[C@H]1OC)C[C@@H](C)[C@@H]2CC(=O)[C@@H](/C=C(/[C@H]([C@H](C(=O)[C@@H](C[C@@H](/C=C/C=C/C=C(/[C@H](C[C@@H]3CC[C@H]([C@@](O3)(C(=O)C(=O)N4CCCC[C@H]4C(=O)O2)O)C)OC)\C)C)C)OC)O)\C)C | |
Source | PubChem | |
URL | https://pubchem.ncbi.nlm.nih.gov | |
Description | Data deposited in or computed by PubChem | |
Molecular Formula |
C55H87NO14 | |
Source | PubChem | |
URL | https://pubchem.ncbi.nlm.nih.gov | |
Description | Data deposited in or computed by PubChem | |
Molecular Weight |
986.3 g/mol | |
Source | PubChem | |
URL | https://pubchem.ncbi.nlm.nih.gov | |
Description | Data deposited in or computed by PubChem | |
Product Name |
Umirolimus | |
CAS RN |
851536-75-9 | |
Record name | Biolimus A9 | |
Source | CAS Common Chemistry | |
URL | https://commonchemistry.cas.org/detail?cas_rn=851536-75-9 | |
Description | CAS Common Chemistry is an open community resource for accessing chemical information. Nearly 500,000 chemical substances from CAS REGISTRY cover areas of community interest, including common and frequently regulated chemicals, and those relevant to high school and undergraduate chemistry classes. This chemical information, curated by our expert scientists, is provided in alignment with our mission as a division of the American Chemical Society. | |
Explanation | The data from CAS Common Chemistry is provided under a CC-BY-NC 4.0 license, unless otherwise stated. | |
Record name | Umirolimus [USAN:INN] | |
Source | ChemIDplus | |
URL | https://pubchem.ncbi.nlm.nih.gov/substance/?source=chemidplus&sourceid=0851536759 | |
Description | ChemIDplus is a free, web search system that provides access to the structure and nomenclature authority files used for the identification of chemical substances cited in National Library of Medicine (NLM) databases, including the TOXNET system. | |
Record name | Umirolimus | |
Source | DrugBank | |
URL | https://www.drugbank.ca/drugs/DB15527 | |
Description | The DrugBank database is a unique bioinformatics and cheminformatics resource that combines detailed drug (i.e. chemical, pharmacological and pharmaceutical) data with comprehensive drug target (i.e. sequence, structure, and pathway) information. | |
Explanation | Creative Common's Attribution-NonCommercial 4.0 International License (http://creativecommons.org/licenses/by-nc/4.0/legalcode) | |
Record name | UMIROLIMUS | |
Source | FDA Global Substance Registration System (GSRS) | |
URL | https://gsrs.ncats.nih.gov/ginas/app/beta/substances/U36PGF65JH | |
Description | The FDA Global Substance Registration System (GSRS) enables the efficient and accurate exchange of information on what substances are in regulated products. Instead of relying on names, which vary across regulatory domains, countries, and regions, the GSRS knowledge base makes it possible for substances to be defined by standardized, scientific descriptions. | |
Explanation | Unless otherwise noted, the contents of the FDA website (www.fda.gov), both text and graphics, are not copyrighted. They are in the public domain and may be republished, reprinted and otherwise used freely by anyone without the need to obtain permission from FDA. Credit to the U.S. Food and Drug Administration as the source is appreciated but not required. | |
Retrosynthesis Analysis
AI-Powered Synthesis Planning: Our tool employs the Template_relevance Pistachio, Template_relevance Bkms_metabolic, Template_relevance Pistachio_ringbreaker, Template_relevance Reaxys, Template_relevance Reaxys_biocatalysis model, leveraging a vast database of chemical reactions to predict feasible synthetic routes.
One-Step Synthesis Focus: Specifically designed for one-step synthesis, it provides concise and direct routes for your target compounds, streamlining the synthesis process.
Accurate Predictions: Utilizing the extensive PISTACHIO, BKMS_METABOLIC, PISTACHIO_RINGBREAKER, REAXYS, REAXYS_BIOCATALYSIS database, our tool offers high-accuracy predictions, reflecting the latest in chemical research and data.
Strategy Settings
Precursor scoring | Relevance Heuristic |
---|---|
Min. plausibility | 0.01 |
Model | Template_relevance |
Template Set | Pistachio/Bkms_metabolic/Pistachio_ringbreaker/Reaxys/Reaxys_biocatalysis |
Top-N result to add to graph | 6 |
Feasible Synthetic Routes
Q & A
Q1: What is Biolimus A9 and what is its mechanism of action?
A1: Biolimus A9, also known as Umirolimus, is a semi-synthetic derivative of sirolimus, belonging to the rapamycin family of compounds. It acts as an antiproliferative agent primarily targeting coronary smooth muscle cells, thus inhibiting restenosis after coronary stent implantation. Like other rapamycin analogs, Biolimus A9 inhibits the mammalian target of rapamycin (mTOR) pathway. [, , , , , ]
Q2: How does Biolimus A9 interact with mTOR?
A2: Biolimus A9 forms a complex with the intracellular immunophilin FK506-binding protein 12 (FKBP12). This complex then binds to mTOR, a serine/threonine protein kinase, inhibiting its activity. [, , , , , ]
Q3: What are the downstream effects of mTOR inhibition by Biolimus A9?
A3: mTOR inhibition primarily blocks cell cycle progression from the G1 to S phase, effectively hindering the proliferation of smooth muscle cells. This inhibition also affects other cellular processes like protein synthesis and angiogenesis, crucial for neointimal hyperplasia. [, , , , , ]
Q4: How does Biolimus A9's effect on smooth muscle cells differ from its effect on endothelial cells?
A4: While Biolimus A9 effectively inhibits smooth muscle cell proliferation, it demonstrates less impact on endothelial cell growth and function. This selectivity is crucial for allowing re-endothelialization of the stented area, promoting healing and reducing the risk of late stent thrombosis. [, , , ]
Q5: What is the molecular formula and weight of Biolimus A9?
A5: Biolimus A9 has a molecular formula of C59H87NO14 and a molecular weight of 1042.3 g/mol. []
Q6: Does Biolimus A9 have any spectroscopic data available?
A6: Spectroscopic data, such as nuclear magnetic resonance (NMR) and infrared (IR) spectroscopy, are crucial for structural elucidation and confirmation. While the provided research papers do not delve into detailed spectroscopic characterization, this information can be found in relevant chemical databases and publications focusing on the synthesis and characterization of Biolimus A9. []
Q7: How is Biolimus A9 incorporated into coronary stents?
A7: Biolimus A9 is coated onto the surface of coronary stents, usually in combination with a polymer carrier. [, ]
Q8: What types of polymers are used with Biolimus A9 in stent coatings?
A8: Both biodegradable polymers like polylactic acid (PLA) and durable polymers like fluoropolymers have been used in Biolimus A9-eluting stents. [, , , ]
Q9: What is the advantage of using a biodegradable polymer with Biolimus A9?
A9: Biodegradable polymers, like PLA, are designed to be gradually broken down and absorbed by the body after the drug has been released. This potentially reduces long-term inflammation and the risk of late stent thrombosis associated with the presence of permanent polymers. [, , , ]
Q10: Are there any concerns regarding the stability of Biolimus A9 within the stent coating?
A10: The stability of Biolimus A9 within the stent coating is crucial for its controlled release and therapeutic efficacy. The formulation of the coating, including the type of polymer and manufacturing processes, is optimized to ensure drug stability and prevent degradation. [, ]
Q11: Does Biolimus A9 exhibit any catalytic properties?
A11: Biolimus A9 primarily functions as an inhibitor of mTOR, and its catalytic properties have not been extensively studied or reported in the context of coronary stent applications. [, , , , , ]
Q12: Have computational methods been used to study Biolimus A9?
A12: Yes, computational chemistry techniques, such as molecular docking and molecular dynamics simulations, can be used to study the interaction of Biolimus A9 with its target, FKBP12, and to understand its binding affinity and selectivity. Additionally, quantitative structure-activity relationship (QSAR) models can be developed to predict the activity of Biolimus A9 analogs based on their structural features. []
Q13: How does the structure of Biolimus A9 contribute to its activity and selectivity?
A13: Specific structural features of Biolimus A9, such as the alkoxy-alkyl group replacing hydrogen at position 42-O, contribute to its enhanced lipophilicity, tissue penetration, and longer cellular residence time compared to sirolimus. These modifications contribute to its potent antiproliferative activity and improved pharmacokinetic profile. [, ]
Q14: What formulation strategies are used to improve the stability, solubility, or bioavailability of Biolimus A9 in stent coatings?
A15: The choice of polymer, drug loading, and coating techniques play a crucial role in controlling the release kinetics and bioavailability of Biolimus A9 from the stent. Asymmetric and abluminal coating strategies have been employed to optimize drug delivery to the vessel wall while minimizing systemic exposure. [, , ]
Q15: What are the SHE regulations surrounding the development and use of Biolimus A9-eluting stents?
A16: Stringent Safety, Health, and Environment (SHE) regulations, such as those established by regulatory agencies like the FDA (USA) and EMA (Europe), govern the development, testing, manufacturing, and clinical use of medical devices like drug-eluting stents. These regulations ensure the quality, safety, and efficacy of the device while minimizing potential risks to patients. []
Q16: What is the pharmacokinetic profile of Biolimus A9?
A17: Biolimus A9 exhibits high lipophilicity, rapid local tissue absorption, a long cellular residence time, and a long terminal half-life of approximately 90 hours. These properties contribute to its sustained therapeutic effect and potentially reduce the risk of systemic side effects. [, ]
Q17: How is Biolimus A9 metabolized and excreted?
A18: While detailed metabolic pathways haven't been extensively described within the provided research, Biolimus A9, like other rapamycin analogs, is primarily metabolized by the cytochrome P450 (CYP3A4) enzyme system in the liver, with metabolites primarily excreted in feces. []
Q18: What preclinical models have been used to evaluate the efficacy and safety of Biolimus A9-eluting stents?
A19: Preclinical studies commonly employ animal models, primarily porcine coronary artery models, to evaluate the efficacy and safety of Biolimus A9-eluting stents. These models allow researchers to assess the stent's impact on neointimal hyperplasia, endothelialization, inflammation, and thrombogenicity. [, , , , ]
Q19: What are the key findings from clinical trials investigating Biolimus A9-eluting stents?
A20: Numerous clinical trials, including LEADERS FREE, NOBORI 1, and STEALTH I, have demonstrated that Biolimus A9-eluting stents effectively reduce restenosis rates, target lesion revascularization, and major adverse cardiac events compared to bare-metal stents. Notably, these trials have highlighted the benefit of Biolimus A9-eluting stents, particularly in patients at high bleeding risk, when used with a shorter duration of dual antiplatelet therapy. [, , , , , , , , ]
Q20: Are there any known mechanisms of resistance to Biolimus A9?
A21: While resistance mechanisms haven't been extensively reported within these studies, prolonged exposure to mTOR inhibitors like Biolimus A9 could potentially lead to the development of resistance through various cellular adaptations, including alterations in drug transporters, target protein mutations, or activation of alternative signaling pathways. []
Q21: How does the design of Biolimus A9-eluting stents optimize drug delivery to the target site?
A23: Stent design parameters, such as stent strut thickness, polymer composition, and coating techniques, are carefully optimized to control the rate and duration of Biolimus A9 release. The goal is to achieve effective local drug concentrations within the vessel wall while minimizing systemic exposure and potential side effects. [, , , ]
Q22: Are there any specific biomarkers under investigation for predicting the efficacy or safety of Biolimus A9-eluting stents?
A24: Research on biomarkers for Biolimus A9-eluting stents is ongoing. Potential biomarkers could include inflammatory markers (e.g., C-reactive protein, interleukin-6), markers of endothelial function (e.g., von Willebrand factor), or genetic markers associated with drug metabolism or response. []
Q23: What analytical methods are used to characterize and quantify Biolimus A9?
A25: Common analytical techniques for characterizing Biolimus A9 include high-performance liquid chromatography (HPLC) and liquid chromatography-tandem mass spectrometry (LC-MS/MS) for quantifying drug concentrations in various matrices, such as plasma, tissue, and stent coatings. [, ]
Q24: How do the dissolution properties of Biolimus A9 from the stent coating impact its bioavailability?
A27: The dissolution rate of Biolimus A9 from the stent coating is a critical factor determining its release kinetics and subsequent bioavailability. A faster dissolution rate typically leads to a more rapid release of the drug into the surrounding tissue. []
Q25: What are the essential parameters for validating analytical methods used to quantify Biolimus A9?
A28: Analytical method validation ensures the accuracy, precision, specificity, linearity, range, detection limit, quantitation limit, and robustness of the method. These parameters are essential for generating reliable and reproducible data for Biolimus A9 quantification in various matrices. []
Q26: What quality control measures are implemented during the manufacturing process of Biolimus A9-eluting stents?
A29: Stringent quality control measures are implemented throughout the manufacturing process of Biolimus A9-eluting stents to ensure product quality, consistency, and safety. These measures encompass raw material testing, process validation, in-process controls, finished product testing, and post-market surveillance. The goal is to minimize batch-to-batch variability and prevent the release of defective products. []
Q27: Does Biolimus A9 elicit an immune response?
A30: While Biolimus A9 is designed to have minimal immunogenicity, its potential to trigger an immune response, though generally low, cannot be completely ruled out. Factors influencing immunogenicity include the individual patient's immune status, drug formulation, and route of administration. []
Q28: Does Biolimus A9 interact with drug transporters, and how might this impact its pharmacokinetics?
A31: Biolimus A9 is a substrate for drug transporters, such as P-glycoprotein (P-gp) and multidrug resistance-associated proteins (MRPs), which play a role in drug absorption, distribution, and excretion. Interactions with these transporters can impact its bioavailability and tissue distribution. []
Q29: Can Biolimus A9 affect the activity of drug-metabolizing enzymes?
A32: Biolimus A9, like other rapamycin analogs, is primarily metabolized by CYP3A4 and can potentially inhibit this enzyme. Co-administration with other drugs that are also metabolized by or induce CYP3A4 might lead to drug interactions and alter their pharmacokinetic profiles. []
Q30: How compatible is Biolimus A9 with biological systems, and what is its biodegradation profile?
A33: Biolimus A9 is designed to be biocompatible, meaning it should not cause significant harm to living tissue. Its biodegradation profile, which refers to its breakdown in the body, is an important consideration for its long-term safety. []
Q31: What are some alternatives to Biolimus A9-eluting stents?
A34: Several other drug-eluting stents are available on the market, each with its own drug, polymer, and elution profile. Common alternatives include stents eluting sirolimus, everolimus, zotarolimus, and paclitaxel. The choice of stent depends on factors such as patient characteristics, lesion complexity, and bleeding risk. [, , ]
Q32: Are there any specific strategies for recycling or managing waste associated with Biolimus A9-eluting stents?
A35: Currently, no established methods for recycling Biolimus A9-eluting stents exist. Therefore, proper disposal according to biomedical waste guidelines is essential to prevent environmental contamination. []
Q33: What research infrastructure and resources are essential for studying Biolimus A9-eluting stents?
A36: Essential resources include access to preclinical animal models, clinical trial networks, specialized imaging equipment (e.g., optical coherence tomography, intravascular ultrasound), and analytical tools for drug quantification and characterization. Collaborative efforts between clinicians, engineers, and basic scientists are crucial for advancing the field. []
Q34: What are some key historical milestones in the development and use of Biolimus A9-eluting stents?
A37: The development of Biolimus A9-eluting stents represents a significant advancement in the field of interventional cardiology. Key milestones include the initial synthesis and characterization of Biolimus A9, preclinical studies demonstrating its efficacy in animal models, and subsequent clinical trials leading to its regulatory approval and widespread clinical use. []
Q35: Are there any potential cross-disciplinary applications for Biolimus A9 or its analogs?
A38: Beyond its use in coronary stents, the potent antiproliferative and immunosuppressive properties of Biolimus A9 and other rapamycin analogs have sparked interest in their potential for other medical applications. These include drug-coated balloons for treating peripheral artery disease, treatment of restenosis in other vascular beds, prevention of transplant rejection, and even potential applications in cancer therapy. []
Disclaimer and Information on In-Vitro Research Products
Please be aware that all articles and product information presented on BenchChem are intended solely for informational purposes. The products available for purchase on BenchChem are specifically designed for in-vitro studies, which are conducted outside of living organisms. In-vitro studies, derived from the Latin term "in glass," involve experiments performed in controlled laboratory settings using cells or tissues. It is important to note that these products are not categorized as medicines or drugs, and they have not received approval from the FDA for the prevention, treatment, or cure of any medical condition, ailment, or disease. We must emphasize that any form of bodily introduction of these products into humans or animals is strictly prohibited by law. It is essential to adhere to these guidelines to ensure compliance with legal and ethical standards in research and experimentation.