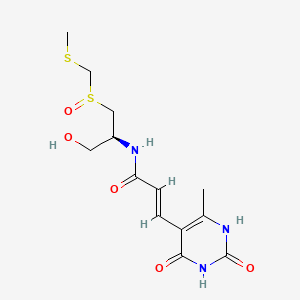
Sparsomycin
Overview
Description
Sparsomycin is a sulfur-containing antitumor antibiotic produced by Streptomyces sparsogenes. It inhibits protein synthesis by binding to the peptidyl transferase center (PTC) of the ribosome, specifically interacting with the universally conserved nucleotide A2602 in the 23S rRNA . Structurally, it features a monooxo-dithioacetal group, a (E)-3-(2,4-dioxo-6-methyl-5-pyrimidinyl)acrylic acid (UCA) moiety, and stereochemical configurations critical for activity (S-configuration at chiral carbons, R-configuration at sulfoxide sulfur) .
Mechanism of Action
Target of Action
Sparsomycin primarily targets the 50S ribosomal subunit . The 50S subunit is a component of the ribosome, the cellular machinery responsible for protein synthesis. By interacting with this subunit, this compound can influence the process of protein synthesis .
Mode of Action
this compound binds to the 50S ribosomal subunit and inhibits protein synthesis through peptidyl transferase inhibition . As it binds to the 50S ribosomal subunit, it induces translocation on the 30S subunit . This interaction disrupts the normal function of the ribosome, leading to changes in protein synthesis.
Biochemical Pathways
The primary biochemical pathway affected by this compound is the protein synthesis pathway . By inhibiting peptidyl transferase, this compound disrupts the formation of peptide bonds, a crucial step in the creation of proteins. This disruption can have downstream effects on various cellular processes that rely on the production of specific proteins.
Result of Action
The primary molecular effect of this compound’s action is the inhibition of protein synthesis . On a cellular level, this can lead to a variety of effects, depending on the specific proteins being synthesized. For example, in tumor cells, the inhibition of protein synthesis could potentially disrupt processes necessary for cell growth and division .
Biochemical Analysis
Biochemical Properties
Sparsomycin interacts with the 50S ribosomal subunit, a component of the ribosome, which is a complex biomolecule responsible for protein synthesis . By binding to the 50S ribosomal subunit, this compound inhibits the process of peptidyl transferase, a critical step in protein synthesis . This interaction disrupts the normal function of the ribosome, leading to inhibition of protein synthesis .
Cellular Effects
The primary cellular effect of this compound is the inhibition of protein synthesis . By binding to the 50S ribosomal subunit, it disrupts the process of protein synthesis, which can have wide-ranging effects on cellular processes . For instance, it can influence cell signaling pathways, gene expression, and cellular metabolism, all of which rely on the proper functioning of protein synthesis .
Molecular Mechanism
This compound exerts its effects at the molecular level primarily through its interaction with the 50S ribosomal subunit . It binds to this subunit and inhibits the process of peptidyl transferase, a critical step in protein synthesis . This binding interaction disrupts the normal function of the ribosome, leading to inhibition of protein synthesis .
Biological Activity
Sparsomycin is an antibiotic compound that has garnered attention due to its unique biological activity, particularly in inhibiting protein synthesis. This article explores its mechanisms, effects on various biological systems, and implications for therapeutic applications.
This compound is classified as a nucleoside antibiotic derived from Streptomyces griseus. Its structure allows it to interfere with the function of ribosomes, the cellular machinery responsible for protein synthesis. Specifically, this compound binds to the 30S ribosomal subunit, inhibiting peptide bond formation during translation. This action leads to the cessation of protein synthesis in susceptible organisms.
Antimicrobial Activity
This compound exhibits broad-spectrum antimicrobial activity against various bacteria and fungi. Its efficacy has been demonstrated in several studies:
- Bacterial Inhibition : this compound shows potent activity against both Gram-positive and Gram-negative bacteria. For instance, it has been effective against Staphylococcus aureus and Escherichia coli.
- Fungal Activity : Research indicates that this compound can inhibit the growth of certain fungi, making it a potential candidate for treating fungal infections.
Antitumor Properties
This compound has also been investigated for its antitumor properties. Studies have shown that it can induce apoptosis in cancer cells through several mechanisms:
- Cell Cycle Arrest : It disrupts the cell cycle progression in various cancer cell lines, leading to increased apoptosis rates.
- Synergistic Effects : When combined with other chemotherapeutic agents, this compound enhances their efficacy, suggesting a potential role in combination therapy for cancer treatment.
Case Study 1: Antitumor Efficacy
A study conducted on human leukemia cell lines demonstrated that this compound significantly reduced cell viability. The findings indicated that treatment with this compound led to a dose-dependent increase in apoptosis markers such as caspase activation and PARP cleavage.
Case Study 2: Antimicrobial Spectrum
In a comparative study of various antibiotics, this compound was found to be particularly effective against multidrug-resistant strains of Staphylococcus aureus. The Minimum Inhibitory Concentration (MIC) values were lower than those for commonly used antibiotics, highlighting its potential as an alternative treatment option.
Data Tables
Q & A
Basic Research Questions
Q. What experimental methodologies are recommended for elucidating sparsomycin’s mechanism of action in ribosomal inhibition?
this compound’s mechanism involves binding to the peptidyl transferase center of ribosomes, inhibiting protein synthesis. To study this, employ biochemical assays like in vitro translation systems with radiolabeled amino acids to track inhibition kinetics. Structural techniques (e.g., cryo-EM or X-ray crystallography) can map binding sites. Validate findings using ribosomal subunits isolated from resistant bacterial strains .
Q. How can researchers optimize the synthesis of this compound analogs for structure-activity relationship (SAR) studies?
Use solid-phase peptide synthesis (SPPS) to modify this compound’s core structure, focusing on its diketopiperazine and sulfur-containing moieties. Purify intermediates via HPLC and confirm stereochemistry with NMR and mass spectrometry. Test analogs in ribosomal inhibition assays to correlate structural changes with activity .
Q. What standardized assays are used to evaluate this compound’s antibacterial efficacy in preclinical studies?
Minimum inhibitory concentration (MIC) assays in bacterial cultures (e.g., Staphylococcus aureus) are standard. Combine with time-kill curve analyses to assess bactericidal vs. bacteriostatic effects. Include control antibiotics (e.g., tetracycline) to contextualize potency. Replicate experiments across multiple bacterial strains to account for variability .
Advanced Research Questions
Q. How can researchers address contradictions in this compound’s reported efficacy across different bacterial models?
Conduct a systematic review to identify confounding variables (e.g., strain-specific ribosomal mutations, growth conditions). Use meta-analysis to quantify heterogeneity (I² statistic) and subgroup analyses (e.g., Gram-positive vs. Gram-negative bacteria). Validate hypotheses via targeted experiments controlling for identified variables .
Q. What strategies improve the translational relevance of in vitro this compound data to in vivo models?
Apply physiologically based pharmacokinetic (PBPK) modeling to predict tissue distribution and bioavailability. Validate using murine infection models with pharmacokinetic/pharmacodynamic (PK/PD) profiling. Ensure bacterial load measurements align with in vitro MIC data, adjusting for host immune responses .
Q. How can researchers design experiments to investigate this compound resistance mechanisms in pathogenic bacteria?
Perform whole-genome sequencing of laboratory-evolved resistant strains to identify mutations in ribosomal genes (e.g., 23S rRNA). Use CRISPR interference to validate candidate mutations. Correlate resistance levels with this compound binding affinity via surface plasmon resonance (SPR) assays .
Q. What computational approaches are effective for designing this compound analogs with reduced host cytotoxicity?
Use molecular docking (e.g., AutoDock Vina) to predict interactions between analogs and human ribosomes. Prioritize compounds with lower binding scores. Validate cytotoxicity in mammalian cell lines (e.g., HEK293) via MTT assays. Cross-reference with bacterial efficacy data to balance selectivity .
Q. How should researchers integrate multi-omics data to identify biomarkers predictive of this compound response?
Perform transcriptomics (RNA-seq) and proteomics (LC-MS/MS) on treated vs. untreated bacterial cultures. Use pathway enrichment analysis (e.g., DAVID) to identify dysregulated processes. Validate biomarkers via knockout strains or siRNA silencing in follow-up experiments .
Q. What statistical methods are suitable for analyzing dose-response relationships in this compound combination therapies?
Apply the Chou-Talalay method to calculate combination indices (CI) for synergism/antagonism. Use nonlinear regression (e.g., GraphPad Prism) to model dose-response curves. Report 95% confidence intervals and perform sensitivity analyses to assess robustness .
Q. How can researchers reconcile discrepancies between this compound’s in vitro potency and clinical trial outcomes?
Retrospectively analyze trial data for covariates like patient comorbidities or pharmacokinetic variability. Use in vitro 3D organoid models to simulate human tissue responses. Re-evaluate dosing regimens via Monte Carlo simulations to optimize target attainment .
Q. Methodological Guidance
- Data Contradictions : Compare results with prior studies using tools like PRISMA for systematic reviews. Discuss variability in experimental conditions (e.g., bacterial growth phase, drug exposure time) .
- Hypothesis Testing : Frame questions using FINER criteria (Feasible, Interesting, Novel, Ethical, Relevant). For example, "Does ribosomal mutation X confer this compound resistance in Pseudomonas aeruginosa?" .
- Statistical Reporting : Adopt STROBE guidelines for observational studies. Report effect sizes, p-values, and power calculations to enhance reproducibility .
Comparison with Similar Compounds
Structural Analogues
Octylsparsomycin
- Structure : A hydrophobic derivative with an octyl chain replacing the hydroxyl group at the dithioacetal moiety.
- Activity :
- Limitations: Limited in vivo data, but reduced toxicity is hypothesized due to lower effective doses.
Sparoxomycins A1/A2
- Structure : Pyrimidinylpropanamide derivatives with modified side chains.
- Activity :
Alkyl Analogues (Compounds 5–7)
- Structure : Alkyl chains (e.g., methyl, ethyl) at the dithioacetal position.
- Activity :
Benzyl Derivatives (Series I–III)
- Structure: Replacement of monooxodithioacetal with 4-substituted benzyl/amide groups.
- Activity :
Functional Analogues
Chloramphenicol
- Mechanism : Binds to the A-site of the PTC, inhibiting peptide bond formation.
- Comparison: No cross-resistance with this compound, indicating distinct binding sites . Lower potency in eukaryotic systems (IC50: 10 μM vs. 0.1 μM for this compound) .
Anisomycin
- Mechanism : Competes with aa-tRNA for PTC binding.
- Comparison :
Key Research Findings
Table 1: In Vitro Activity of this compound and Analogues
Table 2: Structural and Mechanistic Differences
Discussion
Structure-Activity Relationships (SAR)
- Stereochemistry : S-configuration at chiral carbons and R-configuration at sulfoxide sulfur are critical for ribosomal binding .
- Hydrophobicity : Increased lipophilicity (e.g., octylthis compound) enhances cellular uptake and potency .
- Functional Groups : The α-sulfur oxygen and UCA moiety are indispensable for PTC interaction .
Clinical Potential
Properties
CAS No. |
1404-64-4 |
---|---|
Molecular Formula |
C13H19N3O5S2 |
Molecular Weight |
361.4 g/mol |
IUPAC Name |
(E)-N-[1-hydroxy-3-[(R)-methylsulfanylmethylsulfinyl]propan-2-yl]-3-(6-methyl-2,4-dioxo-1H-pyrimidin-5-yl)prop-2-enamide |
InChI |
InChI=1S/C13H19N3O5S2/c1-8-10(12(19)16-13(20)14-8)3-4-11(18)15-9(5-17)6-23(21)7-22-2/h3-4,9,17H,5-7H2,1-2H3,(H,15,18)(H2,14,16,19,20)/b4-3+/t9?,23-/m1/s1 |
InChI Key |
XKLZIVIOZDNKEQ-FBKVPXSSSA-N |
SMILES |
CC1=C(C(=O)NC(=O)N1)C=CC(=O)NC(CO)CS(=O)CSC |
Isomeric SMILES |
CC1=C(C(=O)NC(=O)N1)/C=C/C(=O)NC(CO)C[S@@](=O)CSC |
Canonical SMILES |
CC1=C(C(=O)NC(=O)N1)C=CC(=O)NC(CO)CS(=O)CSC |
Appearance |
Solid powder |
Key on ui other cas no. |
1404-64-4 |
Purity |
>98% (or refer to the Certificate of Analysis) |
shelf_life |
>2 years if stored properly |
solubility |
Soluble in DMSO |
storage |
Dry, dark and at 0 - 4 C for short term (days to weeks) or -20 C for long term (months to years). |
Synonyms |
Sparsomycin; U 19183; U-19183; U19183; B 120121L19; B120121L19; Esparsomicina |
Origin of Product |
United States |
Retrosynthesis Analysis
AI-Powered Synthesis Planning: Our tool employs the Template_relevance Pistachio, Template_relevance Bkms_metabolic, Template_relevance Pistachio_ringbreaker, Template_relevance Reaxys, Template_relevance Reaxys_biocatalysis model, leveraging a vast database of chemical reactions to predict feasible synthetic routes.
One-Step Synthesis Focus: Specifically designed for one-step synthesis, it provides concise and direct routes for your target compounds, streamlining the synthesis process.
Accurate Predictions: Utilizing the extensive PISTACHIO, BKMS_METABOLIC, PISTACHIO_RINGBREAKER, REAXYS, REAXYS_BIOCATALYSIS database, our tool offers high-accuracy predictions, reflecting the latest in chemical research and data.
Strategy Settings
Precursor scoring | Relevance Heuristic |
---|---|
Min. plausibility | 0.01 |
Model | Template_relevance |
Template Set | Pistachio/Bkms_metabolic/Pistachio_ringbreaker/Reaxys/Reaxys_biocatalysis |
Top-N result to add to graph | 6 |
Feasible Synthetic Routes
Disclaimer and Information on In-Vitro Research Products
Please be aware that all articles and product information presented on BenchChem are intended solely for informational purposes. The products available for purchase on BenchChem are specifically designed for in-vitro studies, which are conducted outside of living organisms. In-vitro studies, derived from the Latin term "in glass," involve experiments performed in controlled laboratory settings using cells or tissues. It is important to note that these products are not categorized as medicines or drugs, and they have not received approval from the FDA for the prevention, treatment, or cure of any medical condition, ailment, or disease. We must emphasize that any form of bodily introduction of these products into humans or animals is strictly prohibited by law. It is essential to adhere to these guidelines to ensure compliance with legal and ethical standards in research and experimentation.