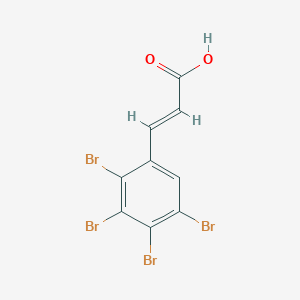
TBCA
Overview
Description
Tribromoisocyanuric acid (TBCA) is a chemical compound widely used in organic synthesis as a brominating agent. It is known for its high efficiency and selectivity in various chemical reactions. This compound is a stable, white crystalline powder with a strong bromine odor. It is often employed in the synthesis of pharmaceuticals, agrochemicals, and other fine chemicals due to its ability to introduce bromine atoms into organic molecules.
Preparation Methods
Synthetic Routes and Reaction Conditions
TBCA can be synthesized through the reaction of cyanuric acid with sodium hydroxide, potassium bromide, and Oxone® in chilled water. The reaction proceeds as follows:
- Dissolve cyanuric acid in a slight molar excess of chilled aqueous sodium hydroxide solution.
- Add potassium bromide and Oxone® to the solution.
- Allow the reaction to proceed at room temperature for 24 hours.
- Filter the resulting product to obtain this compound in excellent yield .
Industrial Production Methods
In industrial settings, this compound is produced using similar methods but on a larger scale. The process involves the use of industrial-grade reagents and equipment to ensure high yield and purity. The reaction conditions are optimized to maximize the efficiency of the synthesis and minimize the production of by-products.
Chemical Reactions Analysis
Types of Reactions
TBCA undergoes various types of chemical reactions, including:
Oxidation: This compound acts as an oxidizing agent in the synthesis of 1,3-diynes via Glaser coupling.
Substitution: This compound is used in the bromination of alkenes, leading to the formation of β-bromoethers, β-bromoacetates, and bromohydrins.
Common Reagents and Conditions
Major Products Formed
Oxidation: 1,4-Diphenylbuta-1,3-diyne.
Substitution: β-Bromoethers, β-bromoacetates, and bromohydrins.
Addition: Vicinal dibromides.
Scientific Research Applications
TBCA has a wide range of applications in scientific research, including:
Mechanism of Action
TBCA exerts its effects primarily through the inhibition of protein kinase CK2. It binds to the ATP-binding site of CK2, preventing the phosphorylation of its substrates. This inhibition leads to the disruption of various cellular processes, including cell proliferation and survival. The molecular targets and pathways involved in this compound’s mechanism of action include the modulation of cytoskeleton integration and cell cycle progression .
Comparison with Similar Compounds
TBCA is often compared to other brominating agents such as:
N-Bromosuccinimide (NBS): This compound has a higher electrophilic character and atom economy compared to NBS, making it more efficient for halogenation reactions.
3,3-Dibromo-5,5-dimethylhydantoin (DBH): This compound is less toxic and less corrosive than DBH, and the by-product cyanuric acid can be reused to produce more this compound.
Trichloroisocyanuric acid (TCCA): This compound is similar to TCCA but is used specifically for bromination reactions, whereas TCCA is used for chlorination.
Conclusion
Tribromoisocyanuric acid (this compound) is a versatile and efficient brominating agent with a wide range of applications in organic synthesis, scientific research, and industry. Its unique properties and high selectivity make it a valuable tool for chemists and researchers. The compound’s ability to inhibit protein kinase CK2 also opens up potential avenues for the development of new therapeutic agents.
Properties
IUPAC Name |
(E)-3-(2,3,4,5-tetrabromophenyl)prop-2-enoic acid | |
---|---|---|
Source | PubChem | |
URL | https://pubchem.ncbi.nlm.nih.gov | |
Description | Data deposited in or computed by PubChem | |
InChI |
InChI=1S/C9H4Br4O2/c10-5-3-4(1-2-6(14)15)7(11)9(13)8(5)12/h1-3H,(H,14,15)/b2-1+ | |
Source | PubChem | |
URL | https://pubchem.ncbi.nlm.nih.gov | |
Description | Data deposited in or computed by PubChem | |
InChI Key |
SVJQCVOKYJWUBC-OWOJBTEDSA-N | |
Source | PubChem | |
URL | https://pubchem.ncbi.nlm.nih.gov | |
Description | Data deposited in or computed by PubChem | |
Canonical SMILES |
C1=C(C(=C(C(=C1Br)Br)Br)Br)C=CC(=O)O | |
Source | PubChem | |
URL | https://pubchem.ncbi.nlm.nih.gov | |
Description | Data deposited in or computed by PubChem | |
Isomeric SMILES |
C1=C(C(=C(C(=C1Br)Br)Br)Br)/C=C/C(=O)O | |
Source | PubChem | |
URL | https://pubchem.ncbi.nlm.nih.gov | |
Description | Data deposited in or computed by PubChem | |
Molecular Formula |
C9H4Br4O2 | |
Source | PubChem | |
URL | https://pubchem.ncbi.nlm.nih.gov | |
Description | Data deposited in or computed by PubChem | |
Molecular Weight |
463.74 g/mol | |
Source | PubChem | |
URL | https://pubchem.ncbi.nlm.nih.gov | |
Description | Data deposited in or computed by PubChem | |
Retrosynthesis Analysis
AI-Powered Synthesis Planning: Our tool employs the Template_relevance Pistachio, Template_relevance Bkms_metabolic, Template_relevance Pistachio_ringbreaker, Template_relevance Reaxys, Template_relevance Reaxys_biocatalysis model, leveraging a vast database of chemical reactions to predict feasible synthetic routes.
One-Step Synthesis Focus: Specifically designed for one-step synthesis, it provides concise and direct routes for your target compounds, streamlining the synthesis process.
Accurate Predictions: Utilizing the extensive PISTACHIO, BKMS_METABOLIC, PISTACHIO_RINGBREAKER, REAXYS, REAXYS_BIOCATALYSIS database, our tool offers high-accuracy predictions, reflecting the latest in chemical research and data.
Strategy Settings
Precursor scoring | Relevance Heuristic |
---|---|
Min. plausibility | 0.01 |
Model | Template_relevance |
Template Set | Pistachio/Bkms_metabolic/Pistachio_ringbreaker/Reaxys/Reaxys_biocatalysis |
Top-N result to add to graph | 6 |
Feasible Synthetic Routes
Q1: What is the molecular formula and weight of TBCA?
A1: The molecular formula of this compound is C9H4Br4O2, and its molecular weight is 483.77 g/mol.
Q2: How does this compound interact with its biological targets?
A2: While the precise mechanism of action is still under investigation, research suggests that this compound inhibits Casein kinase 2 (CK2), an enzyme implicated in cell proliferation and cycle regulation. [] This inhibition leads to significant G2/M phase cell cycle arrest in prostate cancer cell lines. [] Further research is needed to fully elucidate this compound's interactions with CK2 and other potential targets.
Q3: What are the downstream effects of this compound's interaction with CK2?
A3: Inhibition of CK2 by this compound in prostate cancer cells has been shown to significantly reduce cell proliferation and induce G2/M phase cell cycle arrest. [] These effects suggest that this compound may have potential as an anti-cancer agent, but further research is required to confirm this.
Q4: Is there any spectroscopic data available for this compound?
A4: While the provided research papers do not include specific spectroscopic data for this compound, it's characterization likely involves techniques like Fourier-transform infrared spectroscopy (FT-IR) and nuclear magnetic resonance (NMR) spectroscopy. These techniques help confirm the compound's structure and purity.
Q5: Does this compound exhibit any catalytic properties?
A5: While the research provided doesn't focus on catalytic applications of this compound, one study demonstrates the use of a structurally similar compound, tribromoisocyanuric acid (this compound), as an effective reagent for halogenating electron-rich aromatics and carbonyl compounds. [] This suggests that this compound, with its similar structure, might also possess unexplored catalytic potential.
Disclaimer and Information on In-Vitro Research Products
Please be aware that all articles and product information presented on BenchChem are intended solely for informational purposes. The products available for purchase on BenchChem are specifically designed for in-vitro studies, which are conducted outside of living organisms. In-vitro studies, derived from the Latin term "in glass," involve experiments performed in controlled laboratory settings using cells or tissues. It is important to note that these products are not categorized as medicines or drugs, and they have not received approval from the FDA for the prevention, treatment, or cure of any medical condition, ailment, or disease. We must emphasize that any form of bodily introduction of these products into humans or animals is strictly prohibited by law. It is essential to adhere to these guidelines to ensure compliance with legal and ethical standards in research and experimentation.