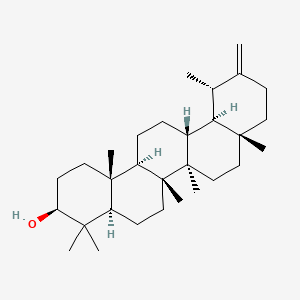
Taraxasterol
Overview
Description
Mechanism of Action
Target of Action
Taraxasterol, a bioactive triterpenoid found in dandelion , primarily targets the EPH receptor A2 (EphA2) and Peroxisome proliferator-activated receptor γ (PPARγ) . These receptors play a crucial role in various diseases, including liver damage, gastritis, colitis, arthritis, pneumonia, tumors, and immune system diseases .
Mode of Action
This compound interacts with its targets to exert significant preventive and therapeutic effects. It inhibits the proliferation of cells, promotes apoptosis, and reduces cell migration and angiogenesis . It also modulates the TLR/NF-κB and Bax/Bc1-2 signaling pathways .
Biochemical Pathways
This compound affects several biochemical pathways. It has been shown to inhibit oxidative stress by increasing the activity of the CYP2E1/Nrf2/HO-1 pathway in animal models of ethanol and high-fat diet-induced liver injury . It also impacts inflammatory action by reducing the levels of inflammatory cytokines, including TNF-α and IL-6, and reduces serum levels of inflammatory mediators NO and PGE₂ through inhibiting NF-κB and MAPK signaling pathways .
Result of Action
The molecular and cellular effects of this compound’s action include significant inhibition of cell proliferation, promotion of apoptosis, and reduction of cell migration and angiogenesis . It also has anti-inflammatory, anti-oxidative, and anti-carcinogenic properties .
Biochemical Analysis
Biochemical Properties
Taraxasterol plays a crucial role in various biochemical reactions. It interacts with several enzymes, proteins, and other biomolecules. One of the key interactions is with the enzyme squalene epoxidase, which catalyzes the cyclization of squalene to 2,3-oxidosqualene, a precursor in the biosynthesis of this compound . Additionally, this compound has been shown to modulate the activity of the CYP2E1/Nrf2/HO-1 pathway, which is involved in oxidative stress responses .
Cellular Effects
This compound exerts significant effects on various cell types and cellular processes. It has been observed to inhibit the inflammatory response in glial cells, potentially protecting against neuronal death in neurodegenerative diseases . In human umbilical vein endothelial cells, this compound reduces the production of inflammatory mediators such as TNF-α, IL-8, PGE2, and NO, and suppresses the expression of iNOS and COX-2 . These actions influence cell signaling pathways, gene expression, and cellular metabolism.
Molecular Mechanism
At the molecular level, this compound exerts its effects through several mechanisms. It activates the peroxisome proliferator-activated receptor γ (PPARγ), which regulates inflammatory signaling pathways by acting on kinases and transcription factors such as NF-κB, c-Jun, and c-Fos . This compound also upregulates the expression of Hint1, a tumor suppressor protein, leading to cell cycle arrest and apoptosis in liver cancer cells .
Temporal Effects in Laboratory Settings
In laboratory settings, the effects of this compound have been observed to change over time. Studies have shown that this compound can inhibit the inflammatory response induced by lipopolysaccharides (LPS) in microglia cells over a 12-hour period The stability and degradation of this compound in vitro and in vivo are crucial for understanding its long-term effects on cellular function
Dosage Effects in Animal Models
The effects of this compound vary with different dosages in animal models. For instance, this compound at doses of 2.5–10 mg/kg has been shown to inhibit oxidative stress by increasing the activity of the CYP2E1/Nrf2/HO-1 pathway in animal models of liver injury . Additionally, this compound dose-dependently attenuated dimethylbenzene-induced mouse ear edema and carrageenan-induced rat paw edema . High doses may lead to toxic or adverse effects, which require careful evaluation.
Metabolic Pathways
This compound is involved in several metabolic pathways. It is derived from the mevalonate pathway, with squalene as a precursor . The biosynthesis involves the cyclization of squalene to 2,3-oxidosqualene, followed by a series of cyclizations and shifts to form the pentacyclic structure of this compound . This compound also influences metabolic flux by inhibiting glycolysis in gastric cancer cells through the downregulation of GPD2 .
Transport and Distribution
Within cells and tissues, this compound is transported and distributed through various mechanisms. It can cross the blood-brain barrier and act directly on glial cells . The compound’s distribution and localization within the cerebrospinal fluid and other tissues are areas of ongoing research. Transporters and binding proteins involved in its distribution are yet to be fully identified.
Subcellular Localization
This compound’s subcellular localization affects its activity and function. It has been found to localize in lipid rafts, disrupting the recruitment of TLR4 into these rafts and inhibiting inflammatory signaling pathways . The acetylation of this compound by triterpene acetyltransferase in lettuce suggests that post-translational modifications may direct it to specific cellular compartments .
Preparation Methods
Synthetic Routes and Reaction Conditions
The biosynthesis of taraxasterol begins with squalene, which undergoes cyclization with molecular oxygen, flavin adenine dinucleotide (FAD), and nicotinamide adenine dinucleotide phosphate (NADPH) via the enzyme squalene epoxidase to yield (2S)-2,3-oxidosqualene . This intermediate is then folded in the chair conformation within the enzyme, leading to a cascade of cyclizations that form the dammarenyl cation. Subsequent alkyl shifts and ring formations result in the tertiary taraxasteryl cation, which is deprotonated to yield this compound .
Industrial Production Methods
Industrial production of this compound typically involves the extraction from dandelion roots, where it is present in significant quantities. The extraction process often includes solvent extraction followed by chromatographic purification to isolate this compound from other triterpenoids and impurities .
Chemical Reactions Analysis
Types of Reactions
Taraxasterol undergoes various chemical reactions, including oxidation, reduction, and substitution reactions.
Common Reagents and Conditions
Oxidation: this compound can be oxidized using reagents such as potassium permanganate or chromium trioxide under acidic conditions to yield corresponding ketones or carboxylic acids.
Reduction: Reduction of this compound can be achieved using hydrogen gas in the presence of a palladium catalyst to form the corresponding alcohols.
Substitution: Halogenation reactions can be performed using halogens like bromine or chlorine in the presence of a catalyst to introduce halogen atoms into the this compound molecule.
Major Products Formed
Oxidation: Formation of ketones or carboxylic acids.
Reduction: Formation of alcohols.
Substitution: Formation of halogenated derivatives.
Scientific Research Applications
Taraxasterol has been extensively studied for its potential therapeutic applications in various fields:
Chemistry: Used as a precursor for synthesizing other bioactive compounds.
Biology: Studied for its role in modulating immune responses and reducing oxidative stress.
Medicine: Investigated for its anti-inflammatory, anti-carcinogenic, and hepatoprotective properties.
Industry: Utilized in the development of pharmaceuticals and nutraceuticals due to its bioactive properties.
Comparison with Similar Compounds
Taraxasterol is unique among triterpenoids due to its specific molecular structure and bioactive properties. Similar compounds include:
Lupeol: Another triterpenoid with anti-inflammatory and anti-carcinogenic properties, but with a different molecular structure.
β-Amyrin: A triterpenoid with similar anti-inflammatory properties but differing in its biosynthetic pathway and molecular structure.
Oleanolic Acid: Known for its hepatoprotective and anti-inflammatory properties, but structurally distinct from this compound.
This compound stands out due to its specific combination of anti-inflammatory, anti-oxidative, and anti-carcinogenic activities, making it a valuable compound for further research and therapeutic applications .
Properties
CAS No. |
1059-14-9 |
---|---|
Molecular Formula |
C30H50O |
Molecular Weight |
426.7 g/mol |
IUPAC Name |
(6aR,6bR,8aR,14bR)-4,4,6a,6b,8a,12,14b-heptamethyl-11-methylidene-1,2,3,4a,5,6,6a,7,8,9,10,12,12a,13,14,14a-hexadecahydropicen-3-ol |
InChI |
InChI=1S/C30H50O/c1-19-11-14-27(5)17-18-29(7)21(25(27)20(19)2)9-10-23-28(6)15-13-24(31)26(3,4)22(28)12-16-30(23,29)8/h20-25,31H,1,9-18H2,2-8H3/t20?,21?,22?,23?,24?,25?,27-,28+,29-,30-/m1/s1 |
InChI Key |
XWMMEBCFHUKHEX-SITXLVLJSA-N |
SMILES |
CC1C2C3CCC4C5(CCC(C(C5CCC4(C3(CCC2(CCC1=C)C)C)C)(C)C)O)C |
Isomeric SMILES |
CC1C2C3CCC4[C@]5(CCC(C(C5CC[C@]4([C@@]3(CC[C@]2(CCC1=C)C)C)C)(C)C)O)C |
Canonical SMILES |
CC1C2C3CCC4C5(CCC(C(C5CCC4(C3(CCC2(CCC1=C)C)C)C)(C)C)O)C |
Appearance |
Solid powder |
melting_point |
225.5 - 226 °C |
physical_description |
Solid |
Purity |
>98% (or refer to the Certificate of Analysis) |
shelf_life |
>3 years if stored properly |
solubility |
Soluble in DMSO |
storage |
Dry, dark and at 0 - 4 C for short term (days to weeks) or -20 C for long term (months to years). |
Synonyms |
Taraxasterol; Isolactucerol |
Origin of Product |
United States |
Retrosynthesis Analysis
AI-Powered Synthesis Planning: Our tool employs the Template_relevance Pistachio, Template_relevance Bkms_metabolic, Template_relevance Pistachio_ringbreaker, Template_relevance Reaxys, Template_relevance Reaxys_biocatalysis model, leveraging a vast database of chemical reactions to predict feasible synthetic routes.
One-Step Synthesis Focus: Specifically designed for one-step synthesis, it provides concise and direct routes for your target compounds, streamlining the synthesis process.
Accurate Predictions: Utilizing the extensive PISTACHIO, BKMS_METABOLIC, PISTACHIO_RINGBREAKER, REAXYS, REAXYS_BIOCATALYSIS database, our tool offers high-accuracy predictions, reflecting the latest in chemical research and data.
Strategy Settings
Precursor scoring | Relevance Heuristic |
---|---|
Min. plausibility | 0.01 |
Model | Template_relevance |
Template Set | Pistachio/Bkms_metabolic/Pistachio_ringbreaker/Reaxys/Reaxys_biocatalysis |
Top-N result to add to graph | 6 |
Feasible Synthetic Routes
Disclaimer and Information on In-Vitro Research Products
Please be aware that all articles and product information presented on BenchChem are intended solely for informational purposes. The products available for purchase on BenchChem are specifically designed for in-vitro studies, which are conducted outside of living organisms. In-vitro studies, derived from the Latin term "in glass," involve experiments performed in controlled laboratory settings using cells or tissues. It is important to note that these products are not categorized as medicines or drugs, and they have not received approval from the FDA for the prevention, treatment, or cure of any medical condition, ailment, or disease. We must emphasize that any form of bodily introduction of these products into humans or animals is strictly prohibited by law. It is essential to adhere to these guidelines to ensure compliance with legal and ethical standards in research and experimentation.