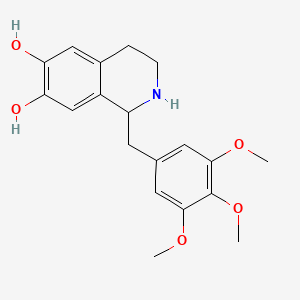
Tretoquinolum
- Click on QUICK INQUIRY to receive a quote from our team of experts.
- With the quality product at a COMPETITIVE price, you can focus more on your research.
Overview
Description
Preparation Methods
Tretoquinol can be synthesized through various synthetic routes. One common method involves the reaction of 3,4,5-trimethoxybenzyl chloride with 6,7-dihydroxy-1,2,3,4-tetrahydroisoquinoline under basic conditions . The reaction typically requires a solvent such as dimethylformamide and a base like potassium carbonate. Industrial production methods may involve similar synthetic routes but are optimized for large-scale production, ensuring high yield and purity of the final product .
Chemical Reactions Analysis
Tretoquinol undergoes several types of chemical reactions, including:
Oxidation: Tretoquinol can be oxidized to form quinone derivatives. Common oxidizing agents include potassium permanganate and hydrogen peroxide.
Reduction: Reduction of tretoquinol can lead to the formation of dihydro derivatives. Sodium borohydride is a typical reducing agent used in these reactions.
Substitution: Tretoquinol can undergo substitution reactions, particularly at the methoxy groups.
Scientific Research Applications
Tretoquinol has a wide range of scientific research applications:
Chemistry: Tretoquinol is used as a model compound in studies of beta-adrenergic agonists and their interactions with receptors.
Biology: It is used in research on cellular signaling pathways and receptor pharmacology.
Medicine: Tretoquinol is studied for its therapeutic potential in treating respiratory diseases such as asthma and chronic obstructive pulmonary disease (COPD).
Industry: It is used in the formulation of pharmaceutical products, particularly those aimed at treating respiratory conditions
Mechanism of Action
Tretoquinol exerts its effects by binding to beta-2 adrenergic receptors on the surface of smooth muscle cells in the airways. This binding activates adenylate cyclase, leading to an increase in cyclic adenosine monophosphate (cAMP) levels. Elevated cAMP levels result in the relaxation of smooth muscle cells, causing bronchodilation and improved airflow . Additionally, tretoquinol can inhibit the release of inflammatory mediators, further contributing to its therapeutic effects .
Comparison with Similar Compounds
Tretoquinol is similar to other beta-2 adrenergic agonists such as salbutamol, terbutaline, and formoterol. tretoquinol is unique in its chemical structure, which includes a trimethoxybenzyl group and a tetrahydroisoquinoline core . This unique structure contributes to its specific pharmacological profile and therapeutic effects. Other similar compounds include:
Salbutamol: A selective beta-2 adrenergic agonist used for the treatment of asthma and COPD.
Terbutaline: Another selective beta-2 adrenergic agonist with similar therapeutic uses.
Formoterol: A long-acting beta-2 adrenergic agonist used for maintenance treatment of asthma and COPD.
Tretoquinol’s distinct structure and pharmacological properties make it a valuable compound in both research and therapeutic applications.
Properties
CAS No. |
30418-38-3 |
---|---|
Molecular Formula |
C19H23NO5 |
Molecular Weight |
345.4 g/mol |
IUPAC Name |
1-[(3,4,5-trimethoxyphenyl)methyl]-1,2,3,4-tetrahydroisoquinoline-6,7-diol |
InChI |
InChI=1S/C19H23NO5/c1-23-17-7-11(8-18(24-2)19(17)25-3)6-14-13-10-16(22)15(21)9-12(13)4-5-20-14/h7-10,14,20-22H,4-6H2,1-3H3 |
InChI Key |
RGVPOXRFEPSFGH-UHFFFAOYSA-N |
Isomeric SMILES |
COC1=CC(=CC(=C1OC)OC)C[C@H]2C3=CC(=C(C=C3CCN2)O)O |
SMILES |
COC1=CC(=CC(=C1OC)OC)CC2C3=CC(=C(C=C3CCN2)O)O |
Canonical SMILES |
COC1=CC(=CC(=C1OC)OC)CC2C3=CC(=C(C=C3CCN2)O)O |
Appearance |
Solid powder |
Purity |
>98% (or refer to the Certificate of Analysis) |
shelf_life |
>2 years if stored properly |
solubility |
Soluble in DMSO |
storage |
Dry, dark and at 0 - 4 C for short term (days to weeks) or -20 C for long term (months to years). |
Synonyms |
AQ 110 AQ-110 AQ110 AQL 208 AQL-208 AQL208 CV 705 CV-705 CV705 Tetroquinol Tretoquinol Tretoquinol Hydrochloride Tretoquinol Hydrochloride Anhydrous Tretoquinol Hydrochloride, (S)-Isomer Tretoquinol-(R) Tretoquinol-(S) HCl Trimethoquinol Trimetoquinol |
Origin of Product |
United States |
Retrosynthesis Analysis
AI-Powered Synthesis Planning: Our tool employs the Template_relevance Pistachio, Template_relevance Bkms_metabolic, Template_relevance Pistachio_ringbreaker, Template_relevance Reaxys, Template_relevance Reaxys_biocatalysis model, leveraging a vast database of chemical reactions to predict feasible synthetic routes.
One-Step Synthesis Focus: Specifically designed for one-step synthesis, it provides concise and direct routes for your target compounds, streamlining the synthesis process.
Accurate Predictions: Utilizing the extensive PISTACHIO, BKMS_METABOLIC, PISTACHIO_RINGBREAKER, REAXYS, REAXYS_BIOCATALYSIS database, our tool offers high-accuracy predictions, reflecting the latest in chemical research and data.
Strategy Settings
Precursor scoring | Relevance Heuristic |
---|---|
Min. plausibility | 0.01 |
Model | Template_relevance |
Template Set | Pistachio/Bkms_metabolic/Pistachio_ringbreaker/Reaxys/Reaxys_biocatalysis |
Top-N result to add to graph | 6 |
Feasible Synthetic Routes
Disclaimer and Information on In-Vitro Research Products
Please be aware that all articles and product information presented on BenchChem are intended solely for informational purposes. The products available for purchase on BenchChem are specifically designed for in-vitro studies, which are conducted outside of living organisms. In-vitro studies, derived from the Latin term "in glass," involve experiments performed in controlled laboratory settings using cells or tissues. It is important to note that these products are not categorized as medicines or drugs, and they have not received approval from the FDA for the prevention, treatment, or cure of any medical condition, ailment, or disease. We must emphasize that any form of bodily introduction of these products into humans or animals is strictly prohibited by law. It is essential to adhere to these guidelines to ensure compliance with legal and ethical standards in research and experimentation.