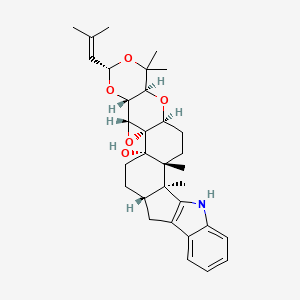
Terpendole C
Overview
Description
Terpendole C is a naturally occurring indole-diterpene compound isolated from fungi, specifically from the genus Albophoma. It is part of a larger family of terpendoles, which are known for their complex molecular structures and significant biological activities. This compound has garnered attention due to its potent inhibitory activity against acyl-CoA: cholesterol acyltransferase (ACAT), an enzyme involved in cholesterol metabolism .
Mechanism of Action
Mode of Action
Terpendole C interacts with its targets, ACAT1 and ACAT2, by inhibiting their activity . This inhibition is potent, with IC50 values of 10 μM for both isozymes . This compound’s most potent inhibitory activity is against cholesteryl ester formation, with an IC50 value of 0.46 μM .
Biochemical Pathways
The inhibition of ACAT by this compound affects the biochemical pathway of cholesteryl ester formation . Cholesteryl esters are crucial components of lipoproteins and play a significant role in the transport of cholesterol in the body. By inhibiting ACAT, this compound disrupts this pathway, potentially affecting cholesterol homeostasis.
Pharmacokinetics
Its molecular weight of 5197 Da suggests that it may have good bioavailability, as molecules under 500 Da are generally well-absorbed
Result of Action
The primary result of this compound’s action is the inhibition of cholesteryl ester formation . This can lead to a decrease in the transport of cholesterol in the body, potentially affecting various cellular processes that rely on cholesterol.
Action Environment
The action of this compound can be influenced by various environmental factors. For instance, the producing organism of this compound, Albophoma yamanashiensis, could potentially affect the compound’s action, efficacy, and stability . Additionally, the physiological environment in which this compound acts, such as pH and temperature, could also influence its activity. More research is needed to fully understand these influences.
Biochemical Analysis
Biochemical Properties
Terpendole C interacts with ACAT1 and ACAT2, inhibiting their activity . These enzymes are involved in the formation of cholesteryl esters, a process that this compound potently inhibits .
Cellular Effects
The inhibition of ACAT1 and ACAT2 by this compound can have significant effects on cellular processes. By inhibiting the formation of cholesteryl esters, this compound can influence cell signaling pathways, gene expression, and cellular metabolism .
Molecular Mechanism
This compound exerts its effects at the molecular level by binding to ACAT1 and ACAT2, inhibiting their activity . This inhibition results in a decrease in the formation of cholesteryl esters .
Temporal Effects in Laboratory Settings
The effects of this compound on ACAT1 and ACAT2 inhibition and cholesteryl ester formation are consistent over time
Metabolic Pathways
This compound is involved in the metabolic pathway of cholesteryl ester formation, where it interacts with the enzymes ACAT1 and ACAT2 . The inhibition of these enzymes by this compound can affect metabolic flux and metabolite levels .
Preparation Methods
Synthetic Routes and Reaction Conditions: The synthesis of terpendole C involves several steps, starting from commercially available starting materials. One of the key synthetic routes includes the construction of the decahydro-1H-benzo[f]chromene system using an intermolecular Diels-Alder reaction. This reaction involves the diene intermediate, 4a-methyl-5-vinyl-3,4,4a,7,8,8a-hexahydro-2H-chromene, and the dienophile, ethyl-3-(1H-indol-2-yl)acrylate .
Industrial Production Methods: Industrial production of this compound is not well-documented, likely due to its complex structure and the challenges associated with its synthesis. advancements in synthetic biology and metabolic engineering could potentially pave the way for more efficient production methods in the future.
Chemical Reactions Analysis
Types of Reactions: Terpendole C undergoes various chemical reactions, including oxidation, reduction, and substitution. These reactions are crucial for modifying its structure and enhancing its biological activity.
Common Reagents and Conditions: Common reagents used in the reactions involving this compound include oxidizing agents like potassium permanganate and reducing agents such as sodium borohydride. The reaction conditions typically involve controlled temperatures and pH levels to ensure the desired transformations.
Major Products Formed: The major products formed from the reactions of this compound depend on the specific reagents and conditions used. For example, oxidation reactions can lead to the formation of hydroxylated derivatives, while reduction reactions can yield deoxygenated compounds.
Scientific Research Applications
Terpendole C has a wide range of scientific research applications due to its biological activities. In chemistry, it serves as a model compound for studying complex natural product synthesis. In biology and medicine, this compound is investigated for its potential as an ACAT inhibitor, which could have implications for treating diseases related to cholesterol metabolism, such as atherosclerosis and fatty liver disease . Additionally, its antifungal and anti-MRSA activities make it a promising candidate for developing new antimicrobial agents .
Comparison with Similar Compounds
Terpendole C is part of a family of indole-diterpenes, which includes other compounds such as terpendole A, terpendole B, and terpendole D. These compounds share a common core structure but differ in their side chains and functional groups, leading to variations in their biological activities. For instance, terpendole D also inhibits ACAT but with slightly lower potency compared to this compound . Other similar compounds include voluhemins, which are also indole-diterpene-containing compounds with inhibitory activity against sterol O-acyltransferase .
Properties
IUPAC Name |
(1R,2S,13S,16S,17S,19R,20R,22S,25S,27S)-1,2,24,24-tetramethyl-22-(2-methylprop-1-enyl)-18,21,23,26-tetraoxa-4-azaoctacyclo[14.13.0.02,13.03,11.05,10.017,19.017,27.020,25]nonacosa-3(11),5,7,9-tetraen-16-ol | |
---|---|---|
Source | PubChem | |
URL | https://pubchem.ncbi.nlm.nih.gov | |
Description | Data deposited in or computed by PubChem | |
InChI |
InChI=1S/C32H41NO5/c1-17(2)15-23-36-24-26(28(3,4)37-23)35-22-12-13-29(5)30(6)18(11-14-31(29,34)32(22)27(24)38-32)16-20-19-9-7-8-10-21(19)33-25(20)30/h7-10,15,18,22-24,26-27,33-34H,11-14,16H2,1-6H3/t18-,22-,23-,24+,26-,27+,29+,30+,31-,32-/m0/s1 | |
Source | PubChem | |
URL | https://pubchem.ncbi.nlm.nih.gov | |
Description | Data deposited in or computed by PubChem | |
InChI Key |
WUOATFFODCBZBE-SCGIUCFSSA-N | |
Source | PubChem | |
URL | https://pubchem.ncbi.nlm.nih.gov | |
Description | Data deposited in or computed by PubChem | |
Canonical SMILES |
CC(=CC1OC2C(C(O1)(C)C)OC3CCC4(C5(C(CCC4(C36C2O6)O)CC7=C5NC8=CC=CC=C78)C)C)C | |
Source | PubChem | |
URL | https://pubchem.ncbi.nlm.nih.gov | |
Description | Data deposited in or computed by PubChem | |
Isomeric SMILES |
CC(=C[C@H]1O[C@H]2[C@@H]3[C@@]4(O3)[C@H](CC[C@]5([C@]4(CC[C@@H]6[C@@]5(C7=C(C6)C8=CC=CC=C8N7)C)O)C)O[C@@H]2C(O1)(C)C)C | |
Source | PubChem | |
URL | https://pubchem.ncbi.nlm.nih.gov | |
Description | Data deposited in or computed by PubChem | |
Molecular Formula |
C32H41NO5 | |
Source | PubChem | |
URL | https://pubchem.ncbi.nlm.nih.gov | |
Description | Data deposited in or computed by PubChem | |
Molecular Weight |
519.7 g/mol | |
Source | PubChem | |
URL | https://pubchem.ncbi.nlm.nih.gov | |
Description | Data deposited in or computed by PubChem | |
Retrosynthesis Analysis
AI-Powered Synthesis Planning: Our tool employs the Template_relevance Pistachio, Template_relevance Bkms_metabolic, Template_relevance Pistachio_ringbreaker, Template_relevance Reaxys, Template_relevance Reaxys_biocatalysis model, leveraging a vast database of chemical reactions to predict feasible synthetic routes.
One-Step Synthesis Focus: Specifically designed for one-step synthesis, it provides concise and direct routes for your target compounds, streamlining the synthesis process.
Accurate Predictions: Utilizing the extensive PISTACHIO, BKMS_METABOLIC, PISTACHIO_RINGBREAKER, REAXYS, REAXYS_BIOCATALYSIS database, our tool offers high-accuracy predictions, reflecting the latest in chemical research and data.
Strategy Settings
Precursor scoring | Relevance Heuristic |
---|---|
Min. plausibility | 0.01 |
Model | Template_relevance |
Template Set | Pistachio/Bkms_metabolic/Pistachio_ringbreaker/Reaxys/Reaxys_biocatalysis |
Top-N result to add to graph | 6 |
Feasible Synthetic Routes
Disclaimer and Information on In-Vitro Research Products
Please be aware that all articles and product information presented on BenchChem are intended solely for informational purposes. The products available for purchase on BenchChem are specifically designed for in-vitro studies, which are conducted outside of living organisms. In-vitro studies, derived from the Latin term "in glass," involve experiments performed in controlled laboratory settings using cells or tissues. It is important to note that these products are not categorized as medicines or drugs, and they have not received approval from the FDA for the prevention, treatment, or cure of any medical condition, ailment, or disease. We must emphasize that any form of bodily introduction of these products into humans or animals is strictly prohibited by law. It is essential to adhere to these guidelines to ensure compliance with legal and ethical standards in research and experimentation.