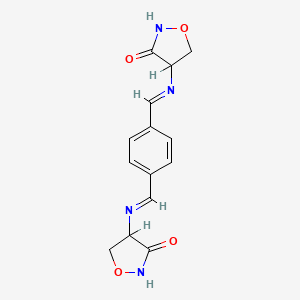
Terizidone
Overview
Description
Terizidone is a pharmaceutical compound primarily used in the treatment of tuberculosis, especially multi-drug-resistant tuberculosis (MDR-TB). It is a derivative of cycloserine and exhibits bacteriostatic properties, meaning it inhibits the growth and reproduction of bacteria rather than killing them directly .
Mechanism of Action
Target of Action
Terizidone, a structural analog of cycloserine, primarily targets two enzymes: L-alanine racemase and D-alanine ligase . These enzymes play a crucial role in bacterial cell wall synthesis. L-alanine racemase converts L-alanine to D-alanine, and D-alanine ligase incorporates D-alanine into the pentapeptide necessary for peptidoglycan formation .
Mode of Action
This compound acts by competitively inhibiting its primary targets, L-alanine racemase and D-alanine ligase . This inhibition disrupts the formation of the alanyl-alanine dipeptide, a critical component of the peptidoglycan layer in the bacterial cell wall . As a result, the integrity of the bacterial cell wall is compromised, leading to bacterial cell death.
Biochemical Pathways
The biochemical pathway affected by this compound is the peptidoglycan synthesis pathway. By inhibiting the enzymes involved in the formation of the alanyl-alanine dipeptide, this compound disrupts the synthesis of peptidoglycan, a major component of the bacterial cell wall . This disruption affects the structural integrity of the bacterial cell wall, leading to cell lysis and death.
Pharmacokinetics
The bioequivalence study should be conducted in the fasting state as this compound can be taken irrespective of meals . For patients with creatinine clearance < 30 ml/min or for patients on haemodialysis, the recommended dose is 250 mg once daily or 500 mg, 3 times per week .
Result of Action
The primary result of this compound’s action is the disruption of bacterial cell wall synthesis, leading to bacterial cell death. This makes this compound effective in the treatment of tuberculosis caused by Mycobacterium tuberculosis .
Action Environment
The efficacy and stability of this compound can be influenced by various environmental factors. For instance, the bioavailability of this compound can be affected by the patient’s renal function, with dose adjustments required for patients with renal failure or those on haemodialysis . Additionally, the start of therapy may be associated with headaches, which can be minimized by starting this compound at lower doses and gradually increasing the dose over one to two weeks .
Biochemical Analysis
Biochemical Properties
Terizidone is a bacteriostatic second-line agent for multi-drug resistant tuberculosis (MDR-TB) used internationally . It is a combination of two molecules of cycloserine . The mechanism of action includes competitively inhibiting l-alanine racemase and d-alanine ligase to inhibit cell wall synthesis .
Cellular Effects
This compound has significant effects on various types of cells and cellular processes. It influences cell function by inhibiting cell wall synthesis . This can impact cell signaling pathways, gene expression, and cellular metabolism.
Molecular Mechanism
This compound exerts its effects at the molecular level through binding interactions with biomolecules. It competitively inhibits l-alanine racemase and d-alanine ligase, enzymes crucial for cell wall synthesis . This inhibition can lead to changes in gene expression and cellular function.
Metabolic Pathways
This compound is involved in metabolic pathways related to the synthesis of cell walls. It interacts with enzymes such as l-alanine racemase and d-alanine ligase
Subcellular Localization
Given its mechanism of action, it can be inferred that this compound likely interacts with enzymes located in the bacterial cell wall .
Preparation Methods
Synthetic Routes and Reaction Conditions: The synthesis of terizidone involves the condensation of two molecules of cycloserine with terephthalaldehyde. The reaction typically occurs in an organic solvent under controlled temperature and pH conditions to ensure the formation of the desired product.
Industrial Production Methods: In industrial settings, the production of this compound follows a similar synthetic route but on a larger scale. The process involves the use of high-purity reagents and solvents, along with advanced purification techniques to obtain this compound in its pure form. The final product is then formulated into capsules or tablets for medical use .
Chemical Reactions Analysis
Types of Reactions: Terizidone undergoes several types of chemical reactions, including:
Hydrolysis: this compound can hydrolyze to form cycloserine, which is its active metabolite.
Oxidation and Reduction: These reactions can modify the functional groups within the this compound molecule, potentially altering its pharmacological properties.
Substitution: this compound can participate in substitution reactions where one functional group is replaced by another.
Common Reagents and Conditions:
Hydrolysis: Typically occurs in aqueous solutions under acidic or basic conditions.
Oxidation: Common oxidizing agents include hydrogen peroxide and potassium permanganate.
Reduction: Reducing agents such as sodium borohydride can be used.
Major Products Formed:
Cycloserine: The primary product formed from the hydrolysis of this compound.
Various oxidized or reduced derivatives: Depending on the specific reagents and conditions used.
Scientific Research Applications
Terizidone has several applications in scientific research, including:
Chemistry: Used as a model compound to study the behavior of oxazolidinones and their derivatives.
Biology: Investigated for its effects on bacterial cell wall synthesis and its potential to inhibit bacterial growth.
Medicine: Primarily used in the treatment of MDR-TB, often in combination with other anti-tuberculosis drugs.
Industry: Utilized in the development of new antibiotics and in the study of drug resistance mechanisms.
Comparison with Similar Compounds
Cycloserine: The parent compound from which terizidone is derived. Both compounds share similar mechanisms of action but differ in their pharmacokinetic properties.
Isoniazid: Another anti-tuberculosis drug that inhibits mycolic acid synthesis, a different target compared to this compound.
Ethionamide: Similar to isoniazid, it targets mycolic acid synthesis but is used in cases where resistance to first-line drugs is present.
Uniqueness of this compound: this compound’s uniqueness lies in its dual action as a prodrug of cycloserine and its ability to inhibit bacterial cell wall synthesis through a different mechanism compared to other anti-tuberculosis drugs. This makes it a valuable option in the treatment of MDR-TB .
Properties
IUPAC Name |
4-[[4-[(3-oxo-1,2-oxazolidin-4-yl)iminomethyl]phenyl]methylideneamino]-1,2-oxazolidin-3-one | |
---|---|---|
Source | PubChem | |
URL | https://pubchem.ncbi.nlm.nih.gov | |
Description | Data deposited in or computed by PubChem | |
InChI |
InChI=1S/C14H14N4O4/c19-13-11(7-21-17-13)15-5-9-1-2-10(4-3-9)6-16-12-8-22-18-14(12)20/h1-6,11-12H,7-8H2,(H,17,19)(H,18,20) | |
Source | PubChem | |
URL | https://pubchem.ncbi.nlm.nih.gov | |
Description | Data deposited in or computed by PubChem | |
InChI Key |
ODKYYBOHSVLGNU-UHFFFAOYSA-N | |
Source | PubChem | |
URL | https://pubchem.ncbi.nlm.nih.gov | |
Description | Data deposited in or computed by PubChem | |
Canonical SMILES |
C1C(C(=O)NO1)N=CC2=CC=C(C=C2)C=NC3CONC3=O | |
Source | PubChem | |
URL | https://pubchem.ncbi.nlm.nih.gov | |
Description | Data deposited in or computed by PubChem | |
Molecular Formula |
C14H14N4O4 | |
Source | PubChem | |
URL | https://pubchem.ncbi.nlm.nih.gov | |
Description | Data deposited in or computed by PubChem | |
DSSTOX Substance ID |
DTXSID30865245, DTXSID70905098 | |
Record name | 4,4'-[1,4-Phenylenebis(methanylylideneazanylylidene)]di(1,2-oxazolidin-3-one) | |
Source | EPA DSSTox | |
URL | https://comptox.epa.gov/dashboard/DTXSID30865245 | |
Description | DSSTox provides a high quality public chemistry resource for supporting improved predictive toxicology. | |
Record name | 4,4'-(p-Phenylenebis(methyleneamino))di-(isoxazolidin-3-one) | |
Source | EPA DSSTox | |
URL | https://comptox.epa.gov/dashboard/DTXSID70905098 | |
Description | DSSTox provides a high quality public chemistry resource for supporting improved predictive toxicology. | |
Molecular Weight |
302.29 g/mol | |
Source | PubChem | |
URL | https://pubchem.ncbi.nlm.nih.gov | |
Description | Data deposited in or computed by PubChem | |
CAS No. |
25683-71-0, 6819-23-4 | |
Record name | 4,4′-[1,4-Phenylenebis(methylidynenitrilo)]bis[3-isoxazolidinone] | |
Source | CAS Common Chemistry | |
URL | https://commonchemistry.cas.org/detail?cas_rn=25683-71-0 | |
Description | CAS Common Chemistry is an open community resource for accessing chemical information. Nearly 500,000 chemical substances from CAS REGISTRY cover areas of community interest, including common and frequently regulated chemicals, and those relevant to high school and undergraduate chemistry classes. This chemical information, curated by our expert scientists, is provided in alignment with our mission as a division of the American Chemical Society. | |
Explanation | The data from CAS Common Chemistry is provided under a CC-BY-NC 4.0 license, unless otherwise stated. | |
Record name | Terizidone [INN] | |
Source | ChemIDplus | |
URL | https://pubchem.ncbi.nlm.nih.gov/substance/?source=chemidplus&sourceid=0025683710 | |
Description | ChemIDplus is a free, web search system that provides access to the structure and nomenclature authority files used for the identification of chemical substances cited in National Library of Medicine (NLM) databases, including the TOXNET system. | |
Record name | Terizidone | |
Source | DrugBank | |
URL | https://www.drugbank.ca/drugs/DB12954 | |
Description | The DrugBank database is a unique bioinformatics and cheminformatics resource that combines detailed drug (i.e. chemical, pharmacological and pharmaceutical) data with comprehensive drug target (i.e. sequence, structure, and pathway) information. | |
Explanation | Creative Common's Attribution-NonCommercial 4.0 International License (http://creativecommons.org/licenses/by-nc/4.0/legalcode) | |
Record name | 4,4'-[1,4-Phenylenebis(methanylylideneazanylylidene)]di(1,2-oxazolidin-3-one) | |
Source | EPA DSSTox | |
URL | https://comptox.epa.gov/dashboard/DTXSID30865245 | |
Description | DSSTox provides a high quality public chemistry resource for supporting improved predictive toxicology. | |
Record name | 4,4'-(p-Phenylenebis(methyleneamino))di-(isoxazolidin-3-one) | |
Source | EPA DSSTox | |
URL | https://comptox.epa.gov/dashboard/DTXSID70905098 | |
Description | DSSTox provides a high quality public chemistry resource for supporting improved predictive toxicology. | |
Record name | Terizidone | |
Source | European Chemicals Agency (ECHA) | |
URL | https://echa.europa.eu/substance-information/-/substanceinfo/100.042.882 | |
Description | The European Chemicals Agency (ECHA) is an agency of the European Union which is the driving force among regulatory authorities in implementing the EU's groundbreaking chemicals legislation for the benefit of human health and the environment as well as for innovation and competitiveness. | |
Explanation | Use of the information, documents and data from the ECHA website is subject to the terms and conditions of this Legal Notice, and subject to other binding limitations provided for under applicable law, the information, documents and data made available on the ECHA website may be reproduced, distributed and/or used, totally or in part, for non-commercial purposes provided that ECHA is acknowledged as the source: "Source: European Chemicals Agency, http://echa.europa.eu/". Such acknowledgement must be included in each copy of the material. ECHA permits and encourages organisations and individuals to create links to the ECHA website under the following cumulative conditions: Links can only be made to webpages that provide a link to the Legal Notice page. | |
Record name | TERIZIDONE | |
Source | FDA Global Substance Registration System (GSRS) | |
URL | https://gsrs.ncats.nih.gov/ginas/app/beta/substances/1199LEX5N8 | |
Description | The FDA Global Substance Registration System (GSRS) enables the efficient and accurate exchange of information on what substances are in regulated products. Instead of relying on names, which vary across regulatory domains, countries, and regions, the GSRS knowledge base makes it possible for substances to be defined by standardized, scientific descriptions. | |
Explanation | Unless otherwise noted, the contents of the FDA website (www.fda.gov), both text and graphics, are not copyrighted. They are in the public domain and may be republished, reprinted and otherwise used freely by anyone without the need to obtain permission from FDA. Credit to the U.S. Food and Drug Administration as the source is appreciated but not required. | |
Retrosynthesis Analysis
AI-Powered Synthesis Planning: Our tool employs the Template_relevance Pistachio, Template_relevance Bkms_metabolic, Template_relevance Pistachio_ringbreaker, Template_relevance Reaxys, Template_relevance Reaxys_biocatalysis model, leveraging a vast database of chemical reactions to predict feasible synthetic routes.
One-Step Synthesis Focus: Specifically designed for one-step synthesis, it provides concise and direct routes for your target compounds, streamlining the synthesis process.
Accurate Predictions: Utilizing the extensive PISTACHIO, BKMS_METABOLIC, PISTACHIO_RINGBREAKER, REAXYS, REAXYS_BIOCATALYSIS database, our tool offers high-accuracy predictions, reflecting the latest in chemical research and data.
Strategy Settings
Precursor scoring | Relevance Heuristic |
---|---|
Min. plausibility | 0.01 |
Model | Template_relevance |
Template Set | Pistachio/Bkms_metabolic/Pistachio_ringbreaker/Reaxys/Reaxys_biocatalysis |
Top-N result to add to graph | 6 |
Feasible Synthetic Routes
Q1: What is Terizidone and how does it work?
A1: this compound (TZD) is a prodrug of cycloserine (CS), a second-line antibiotic used in the treatment of multidrug-resistant tuberculosis (MDR-TB). TZD consists of two molecules of CS linked together. [] Once in the body, TZD is metabolized into CS, which then exerts its antibacterial effect by inhibiting two enzymes crucial for Mycobacterium tuberculosis cell wall synthesis: D-alanine racemase and D-alanine ligase. [, ]
Q2: Does this compound have a better safety profile compared to cycloserine?
A2: While this compound was introduced with the aim of improving tolerability compared to cycloserine, the available evidence from meta-analyses suggests no significant difference or only moderately better safety. [] Further research is needed to fully elucidate this aspect.
Q3: What is the molecular formula and weight of this compound?
A3: this compound has the molecular formula C12H14N4O6 and a molecular weight of 310.26 g/mol. []
Q4: How is this compound structurally characterized?
A4: Various analytical techniques are employed for the structural characterization of TZD, including high-performance liquid chromatography (HPLC), mass spectrometry (MS), infrared (IR) spectroscopy, and nuclear magnetic resonance (NMR) spectroscopy. These techniques provide information about the compound's purity, structure, and fragmentation patterns. [, , , ]
Q5: What is known about the pharmacokinetics of this compound and its metabolite cycloserine?
A5: Studies have shown that TZD achieves higher blood concentrations than CS after administration of equivalent doses. [] This suggests that TZD is metabolized into CS more efficiently, potentially leading to higher CS exposure. Research indicates that dose is a key factor influencing the area under the curve (AUC) for cycloserine after TZD administration. []
Q6: Are there any differences in this compound pharmacokinetics in specific patient populations?
A7: Studies show that elderly patients exhibit higher blood concentrations and slower excretion of TZD compared to younger individuals. [] Further research is needed to assess the clinical significance of this finding.
Q7: How does HIV infection and antiretroviral therapy (ART) influence MDR-TB treatment outcomes in patients receiving this compound?
A8: A study investigating this question found that HIV infection and ART did not significantly impact the cure rates of MDR-TB in patients treated with TZD-containing regimens. []
Q8: What are the implications of additional drug resistance beyond XDR-TB in patients receiving this compound?
A12: A meta-analysis demonstrated that XDR-TB patients with additional resistance to other second-line drugs, including TZD, had significantly lower cure rates and higher odds of treatment failure and death. [] This highlights the need for effective treatment strategies and new drugs to combat highly resistant TB strains.
Q9: What analytical methods are used to quantify this compound in pharmaceutical formulations?
A13: Several analytical methods have been developed for TZD quantification, including spectrophotometry, area under the curve (AUC) analysis, first-order derivative spectrophotometry, and HPLC methods. [, ] These methods offer a range of sensitivity and complexity for different analytical purposes.
Q10: Has a stability-indicating method been developed for this compound analysis?
A14: Yes, a stability-indicating RP-HPLC method has been developed and validated for TZD determination. This method allows for the separation and quantification of TZD from its degradation products under various stress conditions. []
Q11: What is the importance of impurity profiling in this compound research?
A15: Impurity profiling helps identify and characterize potential impurities and degradation products that may arise during TZD manufacturing, storage, and degradation. [] This information is crucial for ensuring drug safety and efficacy.
Q12: How is the quality of this compound controlled and assured?
A12: Quality control and assurance measures for TZD involve adhering to strict manufacturing practices, performing rigorous analytical testing, and implementing robust quality management systems throughout the drug development and distribution process.
Q13: What are the potential future applications of this compound in other research areas?
A17: While primarily used for MDR-TB treatment, TZD's structural features and metal-binding properties make it an interesting candidate for exploration in other areas, such as coordination chemistry and the development of novel metal-based drugs. [, ]
Disclaimer and Information on In-Vitro Research Products
Please be aware that all articles and product information presented on BenchChem are intended solely for informational purposes. The products available for purchase on BenchChem are specifically designed for in-vitro studies, which are conducted outside of living organisms. In-vitro studies, derived from the Latin term "in glass," involve experiments performed in controlled laboratory settings using cells or tissues. It is important to note that these products are not categorized as medicines or drugs, and they have not received approval from the FDA for the prevention, treatment, or cure of any medical condition, ailment, or disease. We must emphasize that any form of bodily introduction of these products into humans or animals is strictly prohibited by law. It is essential to adhere to these guidelines to ensure compliance with legal and ethical standards in research and experimentation.