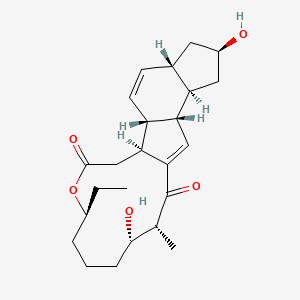
Spinosyn A aglycone
Overview
Description
Spinosyn A aglycone is a naturally derived compound belonging to the spinosyn family of insecticides. These compounds are produced through the fermentation of the bacterium Saccharopolyspora spinosa. This compound is a polyketide-derived tetracyclic macrolide that exhibits potent insecticidal activity against a wide range of pests. It is a key component of the commercial insecticide Spinosad, which is widely used in agriculture and horticulture due to its effectiveness and low environmental impact .
Mechanism of Action
Target of Action
Spinosyn A aglycone primarily targets the nicotinic acetylcholine receptors . These receptors play a crucial role in transmitting signals in the nervous system. By interacting with these receptors, this compound can effectively disrupt the normal functioning of the nervous system in insects .
Mode of Action
The compound’s mode of action involves the disruption of nicotinic acetylcholine receptors . It acts as an allosteric agonist, binding to these receptors and causing a change in their conformation . This change disrupts the normal signaling process, leading to a loss of function in the targeted insects .
Biochemical Pathways
this compound is a product of the polyketide pathway . Its biosynthesis involves the formation of an aglycone from acetate and propionates by polyketide synthase and bridging enzymes . This is followed by glycosylation of the aglycone using deoxythymidine diphosphate (dTDP)-rhamnose, and then methylation of the rhamnose . The resulting compound exhibits potent insecticidal activity .
Pharmacokinetics
It is known that the compound is produced by fermentation , suggesting that it may be metabolized and excreted by biological systems
Result of Action
The result of this compound’s action is potent insecticidal activity . By disrupting the function of nicotinic acetylcholine receptors, it causes paralysis and death in targeted insects . This makes it an effective agent for controlling many commercially significant species that cause extensive damage to crops and other plants .
Action Environment
The action of this compound can be influenced by various environmental factors. It is known that the compound shows potent activity and lower environmental effect, making it a useful agent for modern integrated pest management programs .
Biochemical Analysis
Biochemical Properties
Spinosyn A aglycone plays a crucial role in biochemical reactions, particularly in its interaction with nicotinic acetylcholine receptors. It acts as an allosteric modulator, binding to these receptors and causing prolonged activation, which leads to the disruption of normal neural transmission in insects . The compound interacts with various enzymes and proteins, including polyketide synthases and glycosyltransferases, which are involved in its biosynthesis . These interactions are essential for the formation of the aglycone structure and its subsequent modifications.
Cellular Effects
This compound exerts significant effects on various types of cells and cellular processes. In insect cells, it disrupts neural transmission by binding to nicotinic acetylcholine receptors, leading to paralysis and death . This compound also influences cell signaling pathways, particularly those involved in neurotransmission. Additionally, this compound can affect gene expression and cellular metabolism by altering the activity of key enzymes and transcription factors . These effects are primarily observed in target insect species, with minimal impact on non-target organisms.
Molecular Mechanism
The molecular mechanism of action of this compound involves its binding to nicotinic acetylcholine receptors in the nervous system of insects . This binding causes prolonged activation of the receptors, leading to continuous neural firing and eventual paralysis. At the molecular level, this compound interacts with specific amino acid residues within the receptor, stabilizing its active conformation . This interaction is highly specific, contributing to the compound’s selectivity for insect targets.
Temporal Effects in Laboratory Settings
In laboratory settings, the effects of this compound have been observed to change over time. The compound is relatively stable under standard laboratory conditions, but it can degrade when exposed to light and high temperatures . Long-term studies have shown that this compound can have persistent effects on cellular function, particularly in insect cells. These effects include sustained disruption of neural transmission and prolonged paralysis . The compound’s stability and efficacy can vary depending on the specific experimental conditions.
Dosage Effects in Animal Models
The effects of this compound vary with different dosages in animal models. In insects, low doses of the compound can cause mild neural disruption, while higher doses lead to rapid paralysis and death . Threshold effects have been observed, with a clear dose-response relationship. At high doses, this compound can exhibit toxic effects, including severe neural damage and mortality . These findings highlight the importance of precise dosage control in applications involving this compound.
Metabolic Pathways
This compound is involved in several metabolic pathways, primarily related to its biosynthesis and degradation. The compound is synthesized through the polyketide pathway, involving the action of polyketide synthases and other enzymes . During its biosynthesis, this compound undergoes various modifications, including glycosylation and methylation . These modifications are crucial for the compound’s biological activity and stability. Additionally, this compound can be metabolized by specific enzymes in insect cells, leading to its degradation and elimination .
Transport and Distribution
Within cells and tissues, this compound is transported and distributed through various mechanisms. The compound can interact with specific transporters and binding proteins that facilitate its movement across cellular membranes . Once inside the cell, this compound can accumulate in specific compartments, such as the cytoplasm and the nervous system . This localization is essential for its insecticidal activity, as it allows the compound to reach its target receptors and exert its effects.
Subcellular Localization
The subcellular localization of this compound is primarily within the nervous system of insects. The compound targets nicotinic acetylcholine receptors located on the cell membranes of neurons . This specific localization is facilitated by targeting signals and post-translational modifications that direct this compound to its site of action . The compound’s activity and function are closely linked to its precise localization within the nervous system, which is critical for its insecticidal properties.
Preparation Methods
Synthetic Routes and Reaction Conditions
The preparation of Spinosyn A aglycone involves a complex biosynthetic pathway. The biosynthesis begins with the formation of a linear polyketide chain by polyketide synthases (SpnA-E). This chain undergoes a series of enzymatic reactions, including Diels-Alder and Rauhut-Currier-like reactions, to form the tetracyclic lactone structure of the aglycone . The aglycone is then glycosylated with rhamnose and forosamine to produce the final spinosyn structure .
Industrial Production Methods
Industrial production of this compound is achieved through the fermentation of Saccharopolyspora spinosa. The fermentation process is optimized to maximize the yield of the desired spinosyn compounds. Genetic engineering techniques have been employed to enhance the production of this compound by manipulating the expression of key biosynthetic genes . This approach has led to significant improvements in yield and efficiency.
Chemical Reactions Analysis
Types of Reactions
Spinosyn A aglycone undergoes various chemical reactions, including oxidation, reduction, and substitution. These reactions are essential for the modification and functionalization of the compound to enhance its insecticidal properties.
Common Reagents and Conditions
Common reagents used in the chemical reactions of this compound include oxidizing agents such as potassium permanganate and reducing agents like sodium borohydride. Substitution reactions often involve nucleophiles such as amines and thiols .
Major Products
The major products formed from the chemical reactions of this compound include various spinosyn analogs with modified functional groups. These analogs exhibit different levels of insecticidal activity and are used to target specific pests .
Scientific Research Applications
Spinosyn A aglycone has a wide range of scientific research applications:
Chemistry: It serves as a model compound for studying polyketide biosynthesis and enzymatic reactions.
Biology: Researchers use it to investigate the mechanisms of insecticidal activity and resistance.
Medicine: this compound and its analogs are explored for their potential therapeutic applications, including antiparasitic and antimicrobial properties.
Industry: It is used in the development of environmentally friendly insecticides for integrated pest management programs
Comparison with Similar Compounds
Spinosyn A aglycone is unique among insecticides due to its natural origin, complex structure, and specific mode of action. Similar compounds in the spinosyn family include:
Spinosyn D: A closely related compound with a similar structure and insecticidal activity.
Spinosyn E: A derivative with a demethylated structure.
Spinosyn F: Another derivative with a different demethylation pattern
These compounds share the core tetracyclic macrolide structure but differ in their functional groups and insecticidal properties.
Properties
IUPAC Name |
(1S,2R,5S,7R,9R,10S,14R,15S,19S)-19-ethyl-7,15-dihydroxy-14-methyl-20-oxatetracyclo[10.10.0.02,10.05,9]docosa-3,11-diene-13,21-dione | |
---|---|---|
Source | PubChem | |
URL | https://pubchem.ncbi.nlm.nih.gov | |
Description | Data deposited in or computed by PubChem | |
InChI |
InChI=1S/C24H34O5/c1-3-16-5-4-6-22(26)13(2)24(28)21-11-19-17(20(21)12-23(27)29-16)8-7-14-9-15(25)10-18(14)19/h7-8,11,13-20,22,25-26H,3-6,9-10,12H2,1-2H3/t13-,14-,15-,16+,17-,18-,19-,20+,22+/m1/s1 | |
Source | PubChem | |
URL | https://pubchem.ncbi.nlm.nih.gov | |
Description | Data deposited in or computed by PubChem | |
InChI Key |
DIHUILSVOGYVDG-MRAVEKNJSA-N | |
Source | PubChem | |
URL | https://pubchem.ncbi.nlm.nih.gov | |
Description | Data deposited in or computed by PubChem | |
Canonical SMILES |
CCC1CCCC(C(C(=O)C2=CC3C4CC(CC4C=CC3C2CC(=O)O1)O)C)O | |
Source | PubChem | |
URL | https://pubchem.ncbi.nlm.nih.gov | |
Description | Data deposited in or computed by PubChem | |
Isomeric SMILES |
CC[C@H]1CCC[C@@H]([C@H](C(=O)C2=C[C@H]3[C@@H]4C[C@@H](C[C@H]4C=C[C@H]3[C@@H]2CC(=O)O1)O)C)O | |
Source | PubChem | |
URL | https://pubchem.ncbi.nlm.nih.gov | |
Description | Data deposited in or computed by PubChem | |
Molecular Formula |
C24H34O5 | |
Source | PubChem | |
URL | https://pubchem.ncbi.nlm.nih.gov | |
Description | Data deposited in or computed by PubChem | |
Molecular Weight |
402.5 g/mol | |
Source | PubChem | |
URL | https://pubchem.ncbi.nlm.nih.gov | |
Description | Data deposited in or computed by PubChem | |
Retrosynthesis Analysis
AI-Powered Synthesis Planning: Our tool employs the Template_relevance Pistachio, Template_relevance Bkms_metabolic, Template_relevance Pistachio_ringbreaker, Template_relevance Reaxys, Template_relevance Reaxys_biocatalysis model, leveraging a vast database of chemical reactions to predict feasible synthetic routes.
One-Step Synthesis Focus: Specifically designed for one-step synthesis, it provides concise and direct routes for your target compounds, streamlining the synthesis process.
Accurate Predictions: Utilizing the extensive PISTACHIO, BKMS_METABOLIC, PISTACHIO_RINGBREAKER, REAXYS, REAXYS_BIOCATALYSIS database, our tool offers high-accuracy predictions, reflecting the latest in chemical research and data.
Strategy Settings
Precursor scoring | Relevance Heuristic |
---|---|
Min. plausibility | 0.01 |
Model | Template_relevance |
Template Set | Pistachio/Bkms_metabolic/Pistachio_ringbreaker/Reaxys/Reaxys_biocatalysis |
Top-N result to add to graph | 6 |
Feasible Synthetic Routes
Disclaimer and Information on In-Vitro Research Products
Please be aware that all articles and product information presented on BenchChem are intended solely for informational purposes. The products available for purchase on BenchChem are specifically designed for in-vitro studies, which are conducted outside of living organisms. In-vitro studies, derived from the Latin term "in glass," involve experiments performed in controlled laboratory settings using cells or tissues. It is important to note that these products are not categorized as medicines or drugs, and they have not received approval from the FDA for the prevention, treatment, or cure of any medical condition, ailment, or disease. We must emphasize that any form of bodily introduction of these products into humans or animals is strictly prohibited by law. It is essential to adhere to these guidelines to ensure compliance with legal and ethical standards in research and experimentation.