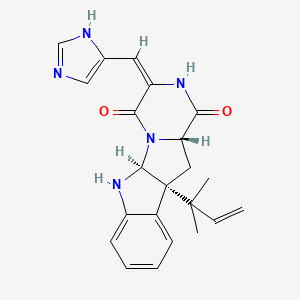
Roquefortine C
Overview
Description
Roquefortine C is a mycotoxin that belongs to a class of naturally occurring 2,5-diketopiperazines. It is produced by various fungi, particularly species from the genus Penicillium. This compound was first isolated from a strain of Penicillium roqueforti, a species commercially used in the maturation of blue-veined cheeses such as Roquefort, Danish Blue, Stilton, and Gorgonzola . This compound is a cyclodipeptide mycotoxin derived from the diketopiperazine cyclo (tryptophan-dehydro-histidine) and is a relatively common fungal metabolite .
Mechanism of Action
Target of Action
Roquefortine C primarily targets mammalian cytochrome P450 enzymes . It also reportedly possesses bacteriostatic activity against gram-positive bacteria , but only in those organisms containing haemoproteins .
Mode of Action
This compound interacts with its targets by inhibiting the growth of gram-positive organisms containing hemins . The mechanisms underlying its toxicity and metabolism have been investigated by studying its interaction with mammalian cytochrome P450 enzymes .
Biochemical Pathways
This compound biosynthesis begins with the condensation of L-tryptophane and L-histidine to form a cyclodipeptide with a diketopiperazine ring by the action of a nonribosomal peptide synthetase (NRPS), named roquefortine dipeptide synthetase (RDS) . This cyclodipeptide is then either dehydrogenated to cyclodehydroHis-Trp or prenylated by the Rpt prenyltransferase to roquefortine D . When the dipeptide cyclodehydroHis-Trp is formed first, the subsequent prenylation reaction forms this compound .
Pharmacokinetics
It is known that this compound is a relatively common fungal metabolite produced by a number of penicillium species . It is soluble in ethanol, methanol, DMF or DMSO .
Result of Action
At high doses, this compound is classified as a toxic compound . Although it is a potent neurotoxin at high doses, at low concentrations of 0.05 to 1.47 mg/kg that occur in domestic cheeses, it was found to be "safe for the consumer" .
Action Environment
This compound is considered one of the most important fungal contaminants of carbonated beverages, beer, wine, meats, cheese, and bread . The production and action of this compound can be influenced by the environment in which the Penicillium species are grown. For example, it was first isolated from a strain of Penicillium roqueforti, a species commercially used as a source of proteolytic and lipolytic enzymes during maturation of the blue-veined cheeses .
Biochemical Analysis
Biochemical Properties
Roquefortine C interacts with various enzymes and proteins. The mechanisms underlying its toxicity and metabolism have been investigated by studying its interaction with mammalian cytochrome P450 enzymes . In addition to these toxic properties, this compound reportedly possesses bacteriostatic activity against gram-positive bacteria, but only in those organisms containing haemoproteins .
Cellular Effects
This compound has significant effects on various types of cells and cellular processes. It is considered one of the most important fungal contaminants of carbonated beverages, beer, wine, meats, cheese, and bread . At high doses, this compound is classified as a toxic compound . Although it is a potent neurotoxin at high doses, at low concentrations of 0.05 to 1.47 mg/kg that occur in domestic cheeses, it was found to be "safe for the consumer" .
Molecular Mechanism
This compound exerts its effects at the molecular level through various mechanisms. It contains the unusual E-dehydrohistidine moiety, a system that typically undergoes facile isomerization under acidic, basic, or photochemical conditions to isothis compound, the 3,12 double-bond Z-isomer of this compound . Isothis compound is not a natural product and in contrast to this compound does not bind iron .
Temporal Effects in Laboratory Settings
The effects of this compound change over time in laboratory settings. Silencing of the rds or rpt genes by the RNAi strategy reduced this compound production by 50% , confirming the involvement of these two key genes in roquefortine biosynthesis.
Dosage Effects in Animal Models
The effects of this compound vary with different dosages in animal models. At high doses, this compound is classified as a toxic compound . Although it is a potent neurotoxin at high doses, at low concentrations of 0.05 to 1.47 mg/kg that occur in domestic cheeses, it was found to be "safe for the consumer" .
Metabolic Pathways
This compound is involved in various metabolic pathways. It is derived from the diketopiperazine cyclo(Trp-dehydro-His) and is a relatively common fungal metabolite produced by a number of Penicillium species .
Preparation Methods
Roquefortine C is primarily produced by Penicillium species through a biosynthetic pathway involving a dimodular nonribosomal peptide synthetase. The biosynthesis begins with the condensation of L-tryptophan and L-histidine to form a cyclodipeptide with a diketopiperazine ring . This cyclodipeptide is then prenylated by the roquefortine prenyltransferase to form roquefortine D, which is subsequently dehydrogenated to this compound by a P450 oxygenase . Industrial production methods involve optimizing the fermentation conditions of Penicillium species to maximize the yield of this compound .
Chemical Reactions Analysis
Roquefortine C undergoes various chemical reactions, including oxidation, reduction, and substitution. It contains an unusual E-dehydrohistidine moiety, which typically undergoes facile isomerization under acidic, basic, or photochemical conditions to form isothis compound . Common reagents and conditions used in these reactions include strong acids, bases, and light exposure. The major products formed from these reactions include isothis compound, which is the 3,12 double-bond Z-isomer of this compound .
Scientific Research Applications
Comparison with Similar Compounds
Roquefortine C is part of a group of prenylated indole alkaloids, which include glandicoline, meleagrin, neoxaline, and acetylaszonalenin . These compounds share a similar biosynthetic pathway and structural features but differ in their specific biological activities and toxicity profiles. For example, meleagrin has been reported to have cytotoxicity against various cancer cell lines, while neoxaline is known for its inhibitory effects on tubulin polymerization . This compound is unique due to its specific interaction with cytochrome P450 enzymes and its bacteriostatic activity against gram-positive bacteria .
Properties
Key on ui mechanism of action |
Roquefortine interaction with rat and human liver cytochromes P450 was monitored by difference UV-vis spectroscopy. It was found to interact with different forms of the cytochromes, giving rise to a type II difference spectrum, characteristic of the binding of an amino function to the heme iron. Roquefortine exhibited high affinity for microsomes from rats treated with various inducers, the K(s) values being in the range 0.2-8 microM. Similar results were observed with human P450 enzymes 1A1, 1A2, 2D6, and 3A4. Roquefortine had no effect on NAPDH cytochrome c reductase. Therefore, inhibition of NADPH consumption was observed using various rat liver microsomes alone or in the presence of 100 microM testosterone in the case of dexamethasone (DEX)-rat microsomes. Enzymatic inhibition was studied in terms of P450 3A activities, i.e., testosterone 6beta-hydroxylase (IC(50) around 10 microM) or bromocriptine metabolism (IC(50) > 50 microM) using DEX-rat liver microsomes or P450 3A4, benzphetamine N-demethylase using phenobarbital-rat liver microsomes (IC(50) > 30 microM), and ethoxyresorufin metabolism using 3-methylcholanthrene-rat liver microsomes (IC(50) 0.1 microM), P450 1A1, and 1A2. Roquefortine was compared with compounds of similar structure: cyclo(Phe-His), cyclo(Phe-dehydroHis), cyclo(Trp-His), phenylahistin. These studies indicate that the =N- imidazole moiety coordinates with the heme iron, and suggest that the dehydroHis moiety and the presence of a fused tetracycle play an important part in roquefortine inhibitory power. |
---|---|
CAS No. |
58735-64-1 |
Molecular Formula |
C22H23N5O2 |
Molecular Weight |
389.4 g/mol |
IUPAC Name |
(4E)-4-(1H-imidazol-5-ylmethylidene)-9-(2-methylbut-3-en-2-yl)-2,5,16-triazatetracyclo[7.7.0.02,7.010,15]hexadeca-10,12,14-triene-3,6-dione |
InChI |
InChI=1S/C22H23N5O2/c1-4-21(2,3)22-10-17-18(28)25-16(9-13-11-23-12-24-13)19(29)27(17)20(22)26-15-8-6-5-7-14(15)22/h4-9,11-12,17,20,26H,1,10H2,2-3H3,(H,23,24)(H,25,28)/b16-9+ |
InChI Key |
SPWSUFUPTSJWNG-CXUHLZMHSA-N |
SMILES |
CC(C)(C=C)C12CC3C(=O)NC(=CC4=CN=CN4)C(=O)N3C1NC5=CC=CC=C25 |
Isomeric SMILES |
CC(C)(C=C)C12CC3C(=O)N/C(=C/C4=CN=CN4)/C(=O)N3C1NC5=CC=CC=C25 |
Canonical SMILES |
CC(C)(C=C)C12CC3C(=O)NC(=CC4=CN=CN4)C(=O)N3C1NC5=CC=CC=C25 |
Appearance |
Solid powder |
melting_point |
202-205 °C 195 - 200 °C |
Key on ui other cas no. |
58735-64-1 |
physical_description |
Solid; [MSDSonline] Solid |
Purity |
>98% (or refer to the Certificate of Analysis) |
shelf_life |
>3 years if stored properly |
solubility |
Soluble in DMSO |
storage |
Dry, dark and at 0 - 4 C for short term (days to weeks) or -20 C for long term (months to years). |
Synonyms |
isoroquefortine C roquefortin roquefortine roquefortine C |
Origin of Product |
United States |
Retrosynthesis Analysis
AI-Powered Synthesis Planning: Our tool employs the Template_relevance Pistachio, Template_relevance Bkms_metabolic, Template_relevance Pistachio_ringbreaker, Template_relevance Reaxys, Template_relevance Reaxys_biocatalysis model, leveraging a vast database of chemical reactions to predict feasible synthetic routes.
One-Step Synthesis Focus: Specifically designed for one-step synthesis, it provides concise and direct routes for your target compounds, streamlining the synthesis process.
Accurate Predictions: Utilizing the extensive PISTACHIO, BKMS_METABOLIC, PISTACHIO_RINGBREAKER, REAXYS, REAXYS_BIOCATALYSIS database, our tool offers high-accuracy predictions, reflecting the latest in chemical research and data.
Strategy Settings
Precursor scoring | Relevance Heuristic |
---|---|
Min. plausibility | 0.01 |
Model | Template_relevance |
Template Set | Pistachio/Bkms_metabolic/Pistachio_ringbreaker/Reaxys/Reaxys_biocatalysis |
Top-N result to add to graph | 6 |
Feasible Synthetic Routes
Disclaimer and Information on In-Vitro Research Products
Please be aware that all articles and product information presented on BenchChem are intended solely for informational purposes. The products available for purchase on BenchChem are specifically designed for in-vitro studies, which are conducted outside of living organisms. In-vitro studies, derived from the Latin term "in glass," involve experiments performed in controlled laboratory settings using cells or tissues. It is important to note that these products are not categorized as medicines or drugs, and they have not received approval from the FDA for the prevention, treatment, or cure of any medical condition, ailment, or disease. We must emphasize that any form of bodily introduction of these products into humans or animals is strictly prohibited by law. It is essential to adhere to these guidelines to ensure compliance with legal and ethical standards in research and experimentation.