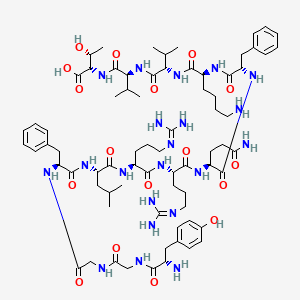
Dynorphin B
Overview
Description
Dynorphin B is a 13-amino acid endogenous opioid peptide derived from the precursor protein prodynorphin. It acts primarily as an agonist of the κ-opioid receptor (KOR), modulating pain perception, stress responses, and motor reflexes in the central and peripheral nervous systems . Unlike other dynorphins (e.g., dynorphin A), this compound exhibits unique functional properties, including lateralized effects on spinal reflexes and complex interactions with opioid antagonists like naloxone . Its distribution in the spinal cord and brain regions such as the hippocampus and nucleus accumbens links it to neuropsychiatric conditions, including chronic pain and depression .
Preparation Methods
Dynorphin B is synthesized through the proteolytic cleavage of leumorphin, which is derived from preproenkephalin B (prodynorphin) . The synthesis involves enzymatic processes where specific enzymes cleave the precursor proteins to produce the active peptide. Industrial production methods typically involve recombinant DNA technology, where the gene encoding preproenkephalin B is inserted into bacterial or yeast cells, which then produce the peptide through fermentation processes .
Chemical Reactions Analysis
Induction of Dynorphin B Release
-
Cannabinoids: Cannabinoid CP55,940 and Δ9-tetrahydrocannabinol (Δ9-THC) can induce the release of this compound, which then acts as an agonist of κ-opioid receptors, resulting in antinociception .
-
TAPS: Tyr-D-Arg-Phe-Sar (TAPS) is capable of promoting the release of this compound through the stimulation of μ1-opioid receptors, also leading to antinociception. The antinociceptive effect produced by this compound allows for spinal analgesia .
Association with Dyskinesia
Studies using imaging mass spectrometry have revealed elevated levels of this compound in the substantia nigra of individuals with L-DOPA induced dyskinesia . The levels of this compound strongly correlate with the severity of dyskinesia . The most significant changes were localized to the lateral part of the substantia nigra .
This compound Variants and Opioid Receptor Selectivity
Several this compound variants with substitutions of naturally occurring amino acids have been investigated for their differential opioid receptor activation . These variants displayed increased selectivity for κ-opioid receptors (KOPrs) over μ-opioid receptors (MOPrs) or δ-opioid receptors (DOPrs) . The increased selectivity for KOPr over MOPr and DOPr was based on a loss of affinity or potency at MOPr and DOPr rather than a higher affinity or potency at KOPr .
Scientific Research Applications
Neuropharmacology and Pain Management
DynB is primarily recognized for its role in modulating pain perception through its action on opioid receptors. Research indicates that DynB has a higher binding affinity for KOPrs compared to mu (MOPrs) and delta opioid receptors (DOPrs), making it a candidate for developing analgesics with fewer side effects associated with traditional opioid therapies.
Case Study: Selectivity for Kappa Opioid Receptors
A recent study investigated various DynB variants to enhance selectivity for KOPr activation while minimizing effects on MOPrs and DOPrs. Seven derivatives exhibited at least a tenfold increase in selectivity for KOPrs, suggesting potential therapeutic applications in pain management without the typical risks of opioid dependency associated with other receptor activations .
Neurological Disorders
DynB has been implicated in several neurological conditions, including epilepsy and Parkinson's disease. Its role in neurotransmission and synaptic plasticity highlights its potential as a therapeutic target.
Case Study: Dyskinesia in Parkinson's Disease
Research utilizing imaging mass spectrometry revealed that elevated levels of DynB correlated with the severity of dyskinesia in patients with Parkinson's disease. This correlation underscores the importance of DynB in the pathophysiology of movement disorders and suggests that targeting DynB could mitigate symptoms associated with dyskinesia .
Addiction and Substance Abuse
DynB plays a critical role in the neurobiological mechanisms underlying addiction, particularly in response to substances like cocaine. Its kappa receptor-independent pathways may provide insights into developing treatments for substance use disorders.
Case Study: Cocaine-Induced Changes
A study found that repeated exposure to cocaine altered the regulation of synaptic transmission mediated by DynB, indicating that DynB's effects persist despite changes induced by drug exposure. This persistence suggests that DynB could be a target for interventions aimed at reducing cocaine-related behaviors .
Mood Disorders
The involvement of DynB in mood regulation has been explored, particularly regarding its potential role in depression. Studies suggest that modulation of dynorphin pathways may yield antidepressant effects.
Case Study: CREB-Dynorphin Pathway
Research demonstrated that antagonism of dynorphins led to antidepressant-like effects in animal models, suggesting that targeting this pathway could provide new avenues for treating depression .
Developmental Biology
DynB also plays a role in developmental processes, particularly concerning cardiomyogenic differentiation. Understanding these mechanisms can provide insights into regenerative medicine and developmental biology.
Case Study: Cardiomyogenic Differentiation
Investigations into the effects of DynB on isolated nuclei have shown that it can elevate gene transcription related to opioid peptides, influencing cardiomyocyte differentiation .
Data Summary Table
Application Area | Key Findings | Implications |
---|---|---|
Neuropharmacology | Increased selectivity for KOPrs among DynB derivatives | Potential for safer analgesics |
Neurological Disorders | Elevated DynB levels correlate with dyskinesia severity | Targeting DynB may alleviate dyskinesia |
Addiction | DynB's role persists despite cocaine exposure | Possible target for addiction treatment |
Mood Disorders | Antagonism of dynorphins yields antidepressant-like effects | New treatment strategies for depression |
Developmental Biology | DynB influences gene transcription related to cardiomyogenic differentiation | Insights into regenerative medicine |
Mechanism of Action
Dynorphin B exerts its effects primarily through the kappa opioid receptor, a G-protein-coupled receptor. Upon binding to the receptor, this compound activates intracellular signaling pathways, leading to the inhibition of adenylate cyclase, reduction of cyclic adenosine monophosphate levels, and modulation of ion channels . This results in the inhibition of neurotransmitter release and the modulation of pain perception .
Comparison with Similar Compounds
Dynorphin A vs. Dynorphin B
Key Findings :
- This compound lacks the C-terminal extension of dynorphin A, reducing its direct binding affinity to KOR but enabling unique interactions with non-opioid pathways (e.g., cannabinoid-induced analgesia) .
α-Neoendorphin vs. This compound
Key Findings :
- α-Neoendorphin exhibits stronger κ-opioid receptor agonism, while this compound’s effects are more context-dependent, involving both excitatory and inhibitory spinal reflexes .
Non-Peptide κ-Opioid Agonists (e.g., U-50488) vs. This compound
Key Findings :
- Synthetic agonists like U-50488 avoid the rapid metabolism and non-opioid interactions seen with this compound, making them preferable for therapeutic use .
Research Implications and Controversies
- Lateral Asymmetry in Spinal Cord: this compound induces side-dependent changes in spinal field potentials (FPs), suggesting uneven κ-opioid receptor distribution across spinal neurons .
- Non-Opioid Mechanisms: this compound’s ability to penetrate cell membranes (unlike dynorphin A) may explain its role in non-canonical signaling pathways, such as cannabinoid-mediated analgesia .
- Species-Specific Effects: Studies in cats show this compound’s lateralized effects on reflexes, absent in rodent models, highlighting interspecies variability .
Biological Activity
Dynorphin B (DynB) is an endogenous opioid peptide derived from the precursor protein prodynorphin. It plays a significant role in various physiological processes, particularly in pain modulation, stress response, and neuroprotection. This article delves into the biological activity of DynB, highlighting its receptor interactions, effects on neural pathways, and implications in disease states.
DynB primarily interacts with kappa-opioid receptors (KORs), exhibiting the highest binding affinity among dynorphins. It also binds to mu-opioid receptors (MOPrs) and delta-opioid receptors (DOPrs) with lower affinity. The activation of KORs by DynB triggers a G-protein-coupled signaling pathway that can lead to various physiological responses, including analgesia and anticonvulsant effects .
Key Findings on Receptor Interaction
- Selectivity for KORs : Recent studies have shown that specific amino acid substitutions in DynB can enhance its selectivity for KORs over MOPrs and DOPrs. For instance, variants like DynB_G3M/Q8H demonstrated a tenfold increase in selectivity for KORs .
- Functional Selectivity : DynB has been observed to preferentially activate the G-protein pathway compared to the β-arrestin pathway, suggesting a complex mechanism of action that may have therapeutic implications .
Biological Effects
DynB's biological effects are diverse and context-dependent, influencing several systems within the body.
Pain Modulation
DynB has been implicated in both analgesic and hyperalgesic responses:
- Analgesia : In animal models, intrathecal administration of DynB has been shown to produce analgesic effects via KOR activation. This effect is often reversible with naloxone, indicating its opioid nature .
- Hyperalgesia : Conversely, certain modifications of DynB can lead to hyperalgesia, particularly when interacting with bradykinin receptors alongside KORs .
Neuroprotective Properties
Research indicates that DynB may play a role in neuroprotection:
- Anticonvulsant Effects : Activation of KORs by DynB has demonstrated anticonvulsant properties, making it a potential target for epilepsy treatment .
- Stress Response : Elevated levels of DynB in the hippocampus have been associated with stress responses and depression models. Blocking KORs can alleviate symptoms of learned helplessness, suggesting a role for DynB in mood regulation .
Case Studies and Experimental Evidence
Several studies have explored the effects of DynB in various contexts:
- Spinal Cord Studies :
- Cardiomyogenic Differentiation :
- Chronic Pain Models :
Research Summary Table
Q & A
Basic Question: What are the standard assays for detecting Dynorphin B in neural tissues, and how can researchers validate their specificity?
Methodological Answer:
this compound detection typically employs immunoassays (e.g., radioimmunoassay, ELISA) or mass spectrometry (LC-MS/MS). Key steps include:
- Tissue Preparation: Use fresh or snap-frozen tissues to prevent peptide degradation. Homogenize in acidic buffers (e.g., 0.1M HCl) to stabilize neuropeptides .
- Antibody Validation: Ensure antibodies are validated via knockout controls or preabsorption with this compound peptides to confirm specificity. Cross-reactivity with similar peptides (e.g., Dynorphin A) must be ruled out .
- Quantitative Analysis: Normalize results to protein concentration or internal standards (e.g., deuterated this compound in LC-MS/MS) .
Advanced Question: How can researchers resolve contradictions in this compound’s κ-opioid receptor (KOR) interactions across different experimental models?
Methodological Answer:
Contradictions often arise from model-specific variables. To address this:
- Model Comparison Table:
Model | Key Variables | Confounding Factors |
---|---|---|
In vitro (cell lines) | Receptor density, coupling efficiency | Non-physiological expression systems |
In vivo (rodents) | Blood-brain barrier penetration | Off-target effects of pharmacological agents |
Human post-mortem | Post-mortem delay, tissue pH | Co-existing neuropathology |
- Unified Protocols: Standardize agonist/antagonist concentrations and use genetic models (e.g., KOR knockout mice) to isolate this compound effects .
- Meta-Analysis: Apply random-effects models to account for heterogeneity across studies .
Basic Question: What experimental design principles ensure reproducibility in this compound release dynamics studies?
Methodological Answer:
- Controlled Stimuli: Use electrical or chemical (e.g., high-K⁺ depolarization) stimuli with calibrated durations to evoke peptide release .
- Temporal Resolution: Employ microdialysis with frequent sampling intervals (5–10 minutes) paired with sensitive detection methods.
- Blinding & Randomization: Assign treatment groups randomly and blind analysts to experimental conditions to reduce bias .
Advanced Question: How should researchers address variability in this compound measurements across imaging and biochemical assays?
Methodological Answer:
- Statistical Harmonization: Use mixed-effects ANOVA to partition variance sources (e.g., assay type, operator skill) .
- Cross-Validation: Correlate imaging data (e.g., GCaMP6M+ fluorescence in neurons) with parallel ELISA measurements in the same tissue samples .
- Power Analysis: Predefine sample sizes using pilot data to ensure adequate power (α=0.05, β=0.2); consult statisticians for complex designs .
Basic Question: What ethical considerations apply to human studies investigating this compound’s role in pain or addiction?
Methodological Answer:
- Informed Consent: Disclose risks of pharmacological interventions (e.g., KOR agonists/ant antagonists) and ensure participant comprehension .
- Data Anonymization: Strip identifiers from datasets, especially in genetic or neuroimaging studies .
- IRB Approval: Submit protocols to institutional review boards, emphasizing safeguards for vulnerable populations (e.g., individuals with substance use disorders) .
Advanced Question: Which statistical approaches are optimal for analyzing this compound’s state-dependent neural activity (e.g., sleep vs. wakefulness)?
Methodological Answer:
- Repeated-Measures ANOVA: Analyze within-subject changes across states (wake, REM, non-REM) to control for individual variability .
- Post-Hoc Corrections: Apply Holm-Sidak tests for pairwise comparisons to reduce Type I error .
- Machine Learning: Use unsupervised clustering (e.g., k-means) to identify activity patterns in large-scale imaging datasets .
Basic Question: How can researchers ensure reproducibility in this compound studies when using animal models?
Methodological Answer:
- Strain Standardization: Use isogenic rodent strains to minimize genetic variability.
- Environmental Controls: Regulate light/dark cycles, diet, and stress exposure across cohorts .
- Open Protocols: Publish detailed methods in supplementary materials, including anesthesia dosages and surgical coordinates for tissue sampling .
Advanced Question: What frameworks (e.g., FINER criteria) guide hypothesis formulation for this compound’s role in neuropsychiatric disorders?
Methodological Answer:
Apply the FINER criteria:
- Feasible: Pilot funding for tissue collection and assay validation.
- Interesting: Link this compound dysregulation to understudied disorders (e.g., comorbid PTSD and addiction).
- Novel: Explore epigenetic regulation of prodynorphin genes.
- Ethical: Use non-invasive imaging in human studies.
- Relevant: Align with NIH priorities on opioid alternatives .
Basic Question: What are best practices for sharing this compound research data to enhance transparency?
Methodological Answer:
- FAIR Principles: Ensure data are Findable (public repositories), Accessible (CC-BY licenses), Interoperable (standardized formats like .csv), and Reusable (metadata templates) .
- Raw Data Inclusion: Provide unprocessed imaging files and chromatograms in supplementary materials .
Advanced Question: How can comparative analysis of this compound across species improve translational relevance?
Methodological Answer:
- Phylogenetic Mapping: Compare prodynorphin sequences in humans, rodents, and non-human primates to identify conserved functional domains.
- Behavioral Paradigms: Use cross-species tasks (e.g., conditioned place aversion) to assess conserved this compound-KOR pathways .
- Dose Equivalency: Calculate allometric scaling factors for translational drug studies .
Properties
IUPAC Name |
(2S,3R)-2-[[(2S)-2-[[(2S)-2-[[(2S)-6-amino-2-[[(2S)-2-[[(2S)-5-amino-2-[[(2S)-2-[[(2S)-2-[[(2S)-2-[[(2S)-2-[[2-[[2-[[(2S)-2-amino-3-(4-hydroxyphenyl)propanoyl]amino]acetyl]amino]acetyl]amino]-3-phenylpropanoyl]amino]-4-methylpentanoyl]amino]-5-(diaminomethylideneamino)pentanoyl]amino]-5-(diaminomethylideneamino)pentanoyl]amino]-5-oxopentanoyl]amino]-3-phenylpropanoyl]amino]hexanoyl]amino]-3-methylbutanoyl]amino]-3-methylbutanoyl]amino]-3-hydroxybutanoic acid | |
---|---|---|
Source | PubChem | |
URL | https://pubchem.ncbi.nlm.nih.gov | |
Description | Data deposited in or computed by PubChem | |
InChI |
InChI=1S/C74H115N21O17/c1-40(2)34-53(91-68(107)54(36-44-18-10-8-11-19-44)86-58(100)39-84-57(99)38-85-62(101)48(76)35-46-25-27-47(97)28-26-46)67(106)89-51(24-17-33-83-74(80)81)63(102)87-50(23-16-32-82-73(78)79)64(103)90-52(29-30-56(77)98)65(104)92-55(37-45-20-12-9-13-21-45)69(108)88-49(22-14-15-31-75)66(105)93-59(41(3)4)70(109)94-60(42(5)6)71(110)95-61(43(7)96)72(111)112/h8-13,18-21,25-28,40-43,48-55,59-61,96-97H,14-17,22-24,29-39,75-76H2,1-7H3,(H2,77,98)(H,84,99)(H,85,101)(H,86,100)(H,87,102)(H,88,108)(H,89,106)(H,90,103)(H,91,107)(H,92,104)(H,93,105)(H,94,109)(H,95,110)(H,111,112)(H4,78,79,82)(H4,80,81,83)/t43-,48+,49+,50+,51+,52+,53+,54+,55+,59+,60+,61+/m1/s1 | |
Source | PubChem | |
URL | https://pubchem.ncbi.nlm.nih.gov | |
Description | Data deposited in or computed by PubChem | |
InChI Key |
AGTSSZRZBSNTGQ-ITZCFHCWSA-N | |
Source | PubChem | |
URL | https://pubchem.ncbi.nlm.nih.gov | |
Description | Data deposited in or computed by PubChem | |
Canonical SMILES |
CC(C)CC(C(=O)NC(CCCN=C(N)N)C(=O)NC(CCCN=C(N)N)C(=O)NC(CCC(=O)N)C(=O)NC(CC1=CC=CC=C1)C(=O)NC(CCCCN)C(=O)NC(C(C)C)C(=O)NC(C(C)C)C(=O)NC(C(C)O)C(=O)O)NC(=O)C(CC2=CC=CC=C2)NC(=O)CNC(=O)CNC(=O)C(CC3=CC=C(C=C3)O)N | |
Source | PubChem | |
URL | https://pubchem.ncbi.nlm.nih.gov | |
Description | Data deposited in or computed by PubChem | |
Isomeric SMILES |
C[C@H]([C@@H](C(=O)O)NC(=O)[C@H](C(C)C)NC(=O)[C@H](C(C)C)NC(=O)[C@H](CCCCN)NC(=O)[C@H](CC1=CC=CC=C1)NC(=O)[C@H](CCC(=O)N)NC(=O)[C@H](CCCN=C(N)N)NC(=O)[C@H](CCCN=C(N)N)NC(=O)[C@H](CC(C)C)NC(=O)[C@H](CC2=CC=CC=C2)NC(=O)CNC(=O)CNC(=O)[C@H](CC3=CC=C(C=C3)O)N)O | |
Source | PubChem | |
URL | https://pubchem.ncbi.nlm.nih.gov | |
Description | Data deposited in or computed by PubChem | |
Molecular Formula |
C74H115N21O17 | |
Source | PubChem | |
URL | https://pubchem.ncbi.nlm.nih.gov | |
Description | Data deposited in or computed by PubChem | |
Molecular Weight |
1570.8 g/mol | |
Source | PubChem | |
URL | https://pubchem.ncbi.nlm.nih.gov | |
Description | Data deposited in or computed by PubChem | |
CAS No. |
83335-41-5 | |
Record name | Rimorphin | |
Source | ChemIDplus | |
URL | https://pubchem.ncbi.nlm.nih.gov/substance/?source=chemidplus&sourceid=0083335415 | |
Description | ChemIDplus is a free, web search system that provides access to the structure and nomenclature authority files used for the identification of chemical substances cited in National Library of Medicine (NLM) databases, including the TOXNET system. | |
Record name | DYNORPHIN B | |
Source | FDA Global Substance Registration System (GSRS) | |
URL | https://gsrs.ncats.nih.gov/ginas/app/beta/substances/K41YC3AEI8 | |
Description | The FDA Global Substance Registration System (GSRS) enables the efficient and accurate exchange of information on what substances are in regulated products. Instead of relying on names, which vary across regulatory domains, countries, and regions, the GSRS knowledge base makes it possible for substances to be defined by standardized, scientific descriptions. | |
Explanation | Unless otherwise noted, the contents of the FDA website (www.fda.gov), both text and graphics, are not copyrighted. They are in the public domain and may be republished, reprinted and otherwise used freely by anyone without the need to obtain permission from FDA. Credit to the U.S. Food and Drug Administration as the source is appreciated but not required. | |
Retrosynthesis Analysis
AI-Powered Synthesis Planning: Our tool employs the Template_relevance Pistachio, Template_relevance Bkms_metabolic, Template_relevance Pistachio_ringbreaker, Template_relevance Reaxys, Template_relevance Reaxys_biocatalysis model, leveraging a vast database of chemical reactions to predict feasible synthetic routes.
One-Step Synthesis Focus: Specifically designed for one-step synthesis, it provides concise and direct routes for your target compounds, streamlining the synthesis process.
Accurate Predictions: Utilizing the extensive PISTACHIO, BKMS_METABOLIC, PISTACHIO_RINGBREAKER, REAXYS, REAXYS_BIOCATALYSIS database, our tool offers high-accuracy predictions, reflecting the latest in chemical research and data.
Strategy Settings
Precursor scoring | Relevance Heuristic |
---|---|
Min. plausibility | 0.01 |
Model | Template_relevance |
Template Set | Pistachio/Bkms_metabolic/Pistachio_ringbreaker/Reaxys/Reaxys_biocatalysis |
Top-N result to add to graph | 6 |
Feasible Synthetic Routes
Disclaimer and Information on In-Vitro Research Products
Please be aware that all articles and product information presented on BenchChem are intended solely for informational purposes. The products available for purchase on BenchChem are specifically designed for in-vitro studies, which are conducted outside of living organisms. In-vitro studies, derived from the Latin term "in glass," involve experiments performed in controlled laboratory settings using cells or tissues. It is important to note that these products are not categorized as medicines or drugs, and they have not received approval from the FDA for the prevention, treatment, or cure of any medical condition, ailment, or disease. We must emphasize that any form of bodily introduction of these products into humans or animals is strictly prohibited by law. It is essential to adhere to these guidelines to ensure compliance with legal and ethical standards in research and experimentation.