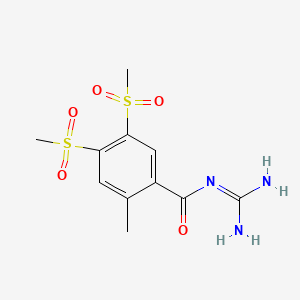
Rimeporide
Overview
Description
Rimeporide (EMD-87580) is a first-in-class sodium/hydrogen exchanger type 1 (NHE-1) inhibitor developed by EspeRare for treating Duchenne Muscular Dystrophy (DMD). Its molecular formula is C₁₁H₁₅N₃O₅S₂ (molecular weight: 333.38 g/mol), and it functions by blocking NHE-1 activity, thereby reducing intracellular sodium and calcium overload and stabilizing pH levels . This mechanism addresses pathological processes in DMD, including muscle edema, inflammation, and fibrosis, which are driven by ion imbalance and oxidative stress .
Preclinical studies in dystrophic animal models (e.g., mdx mice, GRMD dogs) demonstrated this compound’s cardioprotective and anti-fibrotic effects, improving survival rates and cardiac function . A placebo-controlled Phase II trial is planned to validate these findings .
Preparation Methods
The synthetic route for Rimeporide involves the reaction of 2-methyl-4,5-bis(methylsulfonyl)benzoic acid with guanidine under specific conditions. The reaction typically requires a solvent such as dimethylformamide and a catalyst like triethylamine. The product is then purified through crystallization or chromatography .
Chemical Reactions Analysis
Rimeporide undergoes several types of chemical reactions, including:
Oxidation: The compound can be oxidized under specific conditions to form sulfoxides and sulfones.
Reduction: Reduction reactions can convert the sulfone groups back to sulfides.
Substitution: The methylsulfonyl groups can be substituted with other functional groups using appropriate reagents and conditions.
Common reagents used in these reactions include oxidizing agents like hydrogen peroxide, reducing agents like lithium aluminum hydride, and nucleophiles for substitution reactions. The major products formed depend on the specific reaction conditions and reagents used .
Scientific Research Applications
Duchenne Muscular Dystrophy (DMD)
Rimeporide has been repositioned for treating DMD, a genetic disorder characterized by progressive muscle degeneration. The drug's cardioprotective effects have been demonstrated in preclinical models, including dystrophin-deficient mice (mdx) and cardiomyopathic hamsters. Notably, a Phase 1b clinical trial involving 20 boys aged 6-11 years with DMD showed that this compound was safe and well-tolerated, with promising pharmacokinetic and pharmacodynamic outcomes indicating potential cardioprotective benefits .
Key Findings:
- Safety and Tolerability: this compound was well absorbed orally, with no significant adverse effects reported during the trial.
- Efficacy Indicators: Improvements in biomarkers related to cardiac function were observed, supporting further efficacy studies .
Pulmonary Arterial Hypertension (PAH)
Recent studies have explored this compound's effects on PAH, a severe condition marked by high blood pressure in the pulmonary arteries leading to right ventricular failure. In a preclinical model using rats subjected to hypoxia-induced PAH, this compound administration resulted in significant improvements in right ventricular function, reduction of hypertrophy, and attenuation of pulmonary vascular remodeling .
Key Findings:
- Hemodynamic Improvements: this compound improved hemodynamic variables in the pulmonary circulation and decreased right ventricular hypertrophy.
- Biochemical Effects: The treatment led to reduced inflammation and fibrosis in lung tissues .
Comprehensive Data on this compound Applications
Case Study 1: DMD Patient Trial
In a clinical trial involving boys with DMD, this compound was administered over four weeks. The study assessed safety and pharmacodynamic biomarkers. Results indicated that this compound was well tolerated at all doses, with evidence suggesting cardioprotective effects that warrant further investigation .
Case Study 2: PAH Model
In a study using the Sugen5416/hypoxia rat model of PAH, this compound treatment significantly reversed functional impairments associated with the disease. The study demonstrated that this compound not only affected pulmonary hemodynamics but also directly improved right ventricular function by modulating inflammatory pathways .
Mechanism of Action
Rimeporide exerts its effects by inhibiting the sodium/proton exchanger type 1. This inhibition reduces the intracellular concentration of sodium and calcium, which helps in preventing cellular damage and stress. The exact molecular targets and pathways involved include the reduction of pH and ion overload in cells, which is particularly beneficial for patients with Duchenne muscular dystrophy .
Comparison with Similar Compounds
Rimeporide belongs to the NHE-1 inhibitor class, which includes compounds like cariporide, zoniporide, and HOE642. Below is a comparative analysis:
Pharmacological and Structural Properties
- Structural Insights : Acylguanidine derivatives (e.g., this compound, cariporide) exhibit strong NHE-1 binding due to interactions with the transporter’s extracellular domain . This compound’s oral bioavailability distinguishes it from HOE642, which requires injections .
Efficacy in Disease Models
Anti-inflammatory/Anti-fibrotic Effects :
- This compound reduced fibrosis and inflammation in mdx mice and improved cardiac function in DMD models .
- Cariporide showed cardioprotection in ischemia-reperfusion models but failed in clinical trials due to safety issues .
- HOE642 and this compound equally reduced microglial activation and improved motor recovery in stroke models, but only this compound enhanced oligodendrogenesis .
Neuroprotection :
- In murine stroke models, both HOE642 and this compound attenuated neuroinflammation (reduced IBA1+ microglia), but this compound uniquely promoted white matter repair via oligodendrocyte differentiation .
Biological Activity
Rimeporide is a first-in-class sodium/proton exchanger type 1 (NHE-1) inhibitor, primarily investigated for its cardioprotective and anti-inflammatory properties. Originally developed for the treatment of congestive heart failure (CHF), it has garnered attention for its potential applications in Duchenne muscular dystrophy (DMD) and pulmonary arterial hypertension (PAH). This article delves into the biological activity of this compound, highlighting its mechanisms, preclinical and clinical findings, and implications for future therapies.
This compound selectively inhibits NHE-1, a protein that regulates intracellular pH and sodium levels by exchanging intracellular protons for extracellular sodium ions. Under pathological conditions, NHE-1 activity can lead to sodium overload and cellular dysfunction. By inhibiting this exchanger, this compound helps restore ionic balance, thereby exerting protective effects on cardiac and skeletal muscle tissues.
Key Functions of NHE-1 Inhibition:
- Regulation of Intracellular pH : Maintains optimal cellular conditions.
- Prevention of Sodium Overload : Reduces cellular damage associated with excess sodium.
- Anti-inflammatory Effects : Decreases levels of pro-inflammatory cytokines such as IL-6 and TNF-α.
Efficacy in Animal Models
This compound has been extensively studied in various animal models to assess its biological activity:
Notable Research Findings
- A study in mdx mice indicated that this compound significantly reduced creatine kinase levels, a marker of muscle damage, while preserving muscle function.
- In models of PAH, this compound improved hemodynamic parameters and reversed right ventricular hypertrophy.
Phase Ib Study in DMD Patients
A pivotal Phase Ib clinical trial was conducted to evaluate the safety, pharmacokinetics (PK), and pharmacodynamics (PD) of this compound in boys with DMD aged 6 to 14 years. Key outcomes included:
- Safety Profile : this compound was well-tolerated across all doses tested without serious adverse events related to the drug.
- Pharmacokinetics : The drug was efficiently absorbed with linear dose-dependent exposure.
- Biomarkers : Positive exploratory PD biomarkers suggested potential therapeutic benefits in cardiac protection.
Summary of Clinical Findings
Parameter | Outcome |
---|---|
Safety | Well-tolerated; no serious drug-related adverse events reported. |
Pharmacokinetics | Rapid absorption; linear increase in exposure with dose escalation. |
Pharmacodynamics | Encouraging biomarker changes indicating reduced muscle damage and inflammation. |
Case Study: DMD Treatment
In a cohort of 20 boys with DMD treated for four weeks, this compound demonstrated significant improvements in muscle function and reduced markers of inflammation and fibrosis. The results support further investigation into its long-term efficacy as a treatment option for DMD.
Case Study: PAH Management
In a preclinical model of PAH, this compound treatment resulted in notable decreases in right ventricular hypertrophy and pulmonary vascular remodeling, suggesting its potential as a dual-action therapy targeting both pulmonary vasodilation and right ventricular function.
Q & A
Basic Research Questions
Q. What is the pharmacological mechanism of Rimeporide in Duchenne Muscular Dystrophy (DMD), and how is this mechanism investigated preclinically?
this compound is a selective sodium-hydrogen exchanger 1 (NHE-1) inhibitor that modulates intracellular pH and ion homeostasis, addressing pathophysiological mechanisms in DMD such as calcium overload and muscle fibrosis. Preclinical studies in dystrophin-deficient (mdx) mice demonstrated its ability to reduce skeletal muscle necrosis and inflammation. Methodologies include histopathological analysis of muscle tissue, measurement of serum biomarkers (e.g., creatine kinase), and functional assessments (e.g., grip strength, echocardiography) .
Q. How is this compound’s pharmacokinetic (PK) profile modeled in DMD patients, and what covariates are critical for population PK analysis?
A three-compartment model with transit absorption compartments and first-order elimination describes this compound’s PK. Covariates such as serum creatinine (reflecting muscle mass loss in DMD) and total bilirubin (hepatic function) significantly influence distribution volume and clearance. For example, serum creatinine levels explain 25% variability in distribution volume between DMD patients and healthy adults. Nonlinear mixed-effects modeling (NONMEM) and visual predictive checks validate the model .
Q. What experimental design considerations are prioritized in Phase Ib clinical trials for this compound in pediatric DMD populations?
Open-label, multiple ascending dose (MAD) designs are used to assess safety, dose proportionality, and pharmacodynamic (PD) biomarkers. Key considerations include weight-based dosing (four cohorts), inclusion of ambulant boys aged 6–11 years, and exploratory PD endpoints like serum microRNAs (e.g., miR-499) and cardiac imaging biomarkers. Pharmacokinetic bridging from adult data ensures dose optimization .
Q. How do biomarkers inform this compound’s therapeutic efficacy in DMD?
Biomarkers such as serum creatinine (muscle mass), cardiac troponin (myocardial injury), and MRI-based muscle fat fraction are integrated into PK/PD models. Longitudinal correlations between drug exposure and biomarker trajectories are analyzed using mixed-effects repeated measures (MERM) models. For example, reductions in miR-499 levels after 4 weeks of treatment suggest cardioprotective effects .
Advanced Research Questions
Q. How can researchers resolve contradictions in this compound’s absorption and clearance parameters across studies?
Discrepancies, such as 50% lower absorption under fed conditions or 20% reduced clearance in low-GFR subgroups, are addressed via covariate-adjusted sensitivity analyses. Physiologically based pharmacokinetic (PBPK) modeling isolates food effects on gastric transit, while Bayesian hierarchical models pool heterogeneous datasets (e.g., adult vs. pediatric) to account for inter-study variability .
Q. What statistical methods validate this compound’s PK-PD relationships in the context of DMD progression?
Disease progression models incorporate time-dependent covariates (e.g., annualized loss of ambulation) to predict long-term exposure changes. Non-parametric bootstrapping quantifies parameter uncertainty, and external validation with muscle biopsy data strengthens model generalizability. Sensitivity analyses adjust for confounders like age and concomitant therapies .
Q. How can preclinical and clinical data on this compound’s skeletal vs. cardiac efficacy be reconciled?
Transcriptomic profiling of mdx mice versus human DMD biopsies identifies conserved NHE-1 signaling pathways. Dose-response meta-regression quantifies interspecies differences in target engagement thresholds. Reverse translation frameworks refine preclinical models (e.g., humanized NHE-1 knock-in mice) to better mimic human pathophysiology .
Q. What strategies optimize this compound’s PK model for non-ambulant DMD populations excluded from initial trials?
Mechanism-based modeling integrates preclinical data from severe cardiomyopathy models (e.g., aged mdx mice) to simulate drug penetration into fibrotic tissue. Virtual twin simulations predict exposure in advanced disease stages, validated against real-world data from registries like TREAT-NMD .
Q. How should researchers address variability in this compound’s distribution volume linked to DMD-related muscle wasting?
Allometric scaling with lean body mass or serum creatinine accounts for reduced muscle mass. Population PK simulations incorporate disease progression metrics (e.g., annualized decline in ambulatory function) to adjust dosing regimens. Covariate analysis identifies subpopulations requiring personalized dosing .
Q. What methodologies validate the cardioprotective effects of this compound in long-term DMD studies?
Multi-modal data fusion combines echocardiography, cardiac MRI, and proteomic profiles to detect early therapeutic effects. Time-to-event analysis evaluates delays in cardiomyopathy onset, while mechanistic PK/PD models correlate drug exposure with reductions in fibrosis biomarkers (e.g., collagen degradation products) .
Properties
IUPAC Name |
N-(diaminomethylidene)-2-methyl-4,5-bis(methylsulfonyl)benzamide | |
---|---|---|
Source | PubChem | |
URL | https://pubchem.ncbi.nlm.nih.gov | |
Description | Data deposited in or computed by PubChem | |
InChI |
InChI=1S/C11H15N3O5S2/c1-6-4-8(20(2,16)17)9(21(3,18)19)5-7(6)10(15)14-11(12)13/h4-5H,1-3H3,(H4,12,13,14,15) | |
Source | PubChem | |
URL | https://pubchem.ncbi.nlm.nih.gov | |
Description | Data deposited in or computed by PubChem | |
InChI Key |
GROMEQPXDKRRIE-UHFFFAOYSA-N | |
Source | PubChem | |
URL | https://pubchem.ncbi.nlm.nih.gov | |
Description | Data deposited in or computed by PubChem | |
Canonical SMILES |
CC1=CC(=C(C=C1C(=O)N=C(N)N)S(=O)(=O)C)S(=O)(=O)C | |
Source | PubChem | |
URL | https://pubchem.ncbi.nlm.nih.gov | |
Description | Data deposited in or computed by PubChem | |
Molecular Formula |
C11H15N3O5S2 | |
Source | PubChem | |
URL | https://pubchem.ncbi.nlm.nih.gov | |
Description | Data deposited in or computed by PubChem | |
DSSTOX Substance ID |
DTXSID30870177 | |
Record name | Rimeporide | |
Source | EPA DSSTox | |
URL | https://comptox.epa.gov/dashboard/DTXSID30870177 | |
Description | DSSTox provides a high quality public chemistry resource for supporting improved predictive toxicology. | |
Molecular Weight |
333.4 g/mol | |
Source | PubChem | |
URL | https://pubchem.ncbi.nlm.nih.gov | |
Description | Data deposited in or computed by PubChem | |
CAS No. |
187870-78-6 | |
Record name | N-(Aminoiminomethyl)-2-methyl-4,5-bis(methylsulfonyl)benzamide | |
Source | CAS Common Chemistry | |
URL | https://commonchemistry.cas.org/detail?cas_rn=187870-78-6 | |
Description | CAS Common Chemistry is an open community resource for accessing chemical information. Nearly 500,000 chemical substances from CAS REGISTRY cover areas of community interest, including common and frequently regulated chemicals, and those relevant to high school and undergraduate chemistry classes. This chemical information, curated by our expert scientists, is provided in alignment with our mission as a division of the American Chemical Society. | |
Explanation | The data from CAS Common Chemistry is provided under a CC-BY-NC 4.0 license, unless otherwise stated. | |
Record name | Rimeporide [INN] | |
Source | ChemIDplus | |
URL | https://pubchem.ncbi.nlm.nih.gov/substance/?source=chemidplus&sourceid=0187870786 | |
Description | ChemIDplus is a free, web search system that provides access to the structure and nomenclature authority files used for the identification of chemical substances cited in National Library of Medicine (NLM) databases, including the TOXNET system. | |
Record name | Rimeporide | |
Source | DrugBank | |
URL | https://www.drugbank.ca/drugs/DB12861 | |
Description | The DrugBank database is a unique bioinformatics and cheminformatics resource that combines detailed drug (i.e. chemical, pharmacological and pharmaceutical) data with comprehensive drug target (i.e. sequence, structure, and pathway) information. | |
Explanation | Creative Common's Attribution-NonCommercial 4.0 International License (http://creativecommons.org/licenses/by-nc/4.0/legalcode) | |
Record name | Rimeporide | |
Source | EPA DSSTox | |
URL | https://comptox.epa.gov/dashboard/DTXSID30870177 | |
Description | DSSTox provides a high quality public chemistry resource for supporting improved predictive toxicology. | |
Record name | RIMEPORIDE | |
Source | FDA Global Substance Registration System (GSRS) | |
URL | https://gsrs.ncats.nih.gov/ginas/app/beta/substances/QH6B4V5743 | |
Description | The FDA Global Substance Registration System (GSRS) enables the efficient and accurate exchange of information on what substances are in regulated products. Instead of relying on names, which vary across regulatory domains, countries, and regions, the GSRS knowledge base makes it possible for substances to be defined by standardized, scientific descriptions. | |
Explanation | Unless otherwise noted, the contents of the FDA website (www.fda.gov), both text and graphics, are not copyrighted. They are in the public domain and may be republished, reprinted and otherwise used freely by anyone without the need to obtain permission from FDA. Credit to the U.S. Food and Drug Administration as the source is appreciated but not required. | |
Synthesis routes and methods
Procedure details
Retrosynthesis Analysis
AI-Powered Synthesis Planning: Our tool employs the Template_relevance Pistachio, Template_relevance Bkms_metabolic, Template_relevance Pistachio_ringbreaker, Template_relevance Reaxys, Template_relevance Reaxys_biocatalysis model, leveraging a vast database of chemical reactions to predict feasible synthetic routes.
One-Step Synthesis Focus: Specifically designed for one-step synthesis, it provides concise and direct routes for your target compounds, streamlining the synthesis process.
Accurate Predictions: Utilizing the extensive PISTACHIO, BKMS_METABOLIC, PISTACHIO_RINGBREAKER, REAXYS, REAXYS_BIOCATALYSIS database, our tool offers high-accuracy predictions, reflecting the latest in chemical research and data.
Strategy Settings
Precursor scoring | Relevance Heuristic |
---|---|
Min. plausibility | 0.01 |
Model | Template_relevance |
Template Set | Pistachio/Bkms_metabolic/Pistachio_ringbreaker/Reaxys/Reaxys_biocatalysis |
Top-N result to add to graph | 6 |
Feasible Synthetic Routes
Disclaimer and Information on In-Vitro Research Products
Please be aware that all articles and product information presented on BenchChem are intended solely for informational purposes. The products available for purchase on BenchChem are specifically designed for in-vitro studies, which are conducted outside of living organisms. In-vitro studies, derived from the Latin term "in glass," involve experiments performed in controlled laboratory settings using cells or tissues. It is important to note that these products are not categorized as medicines or drugs, and they have not received approval from the FDA for the prevention, treatment, or cure of any medical condition, ailment, or disease. We must emphasize that any form of bodily introduction of these products into humans or animals is strictly prohibited by law. It is essential to adhere to these guidelines to ensure compliance with legal and ethical standards in research and experimentation.