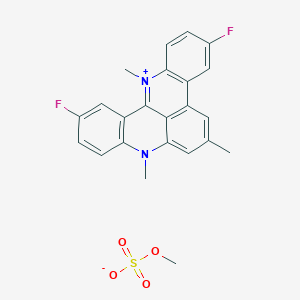
RHPS4
Overview
Description
RHPS4 (3,11-difluoro-6,8,13-trimethyl-8H-quino[4,3,2-kl]acridinium methosulfate) is a pentacyclic acridine derivative and a potent G-quadruplex (G4) stabilizing ligand. It selectively binds to and stabilizes G4 DNA structures, particularly in telomeres, disrupting telomere maintenance and inhibiting telomerase activity. This compound exhibits high anti-proliferative effects in cancer cells by inducing telomere dysfunction, DNA damage responses, and cell cycle arrest (S/G2 phase) . Its IC50 for telomerase inhibition is 0.33 µM in cell-free assays , and it demonstrates synergistic effects with radiotherapy and chemotherapeutics like Taxol in glioblastoma models .
Preparation Methods
The synthesis of RHPS 4 methosulfate involves the formation of the quinoacridinium core followed by the introduction of the methosulfate group. The synthetic route typically includes:
Formation of the quinoacridinium core: This step involves the cyclization of appropriate precursors under acidic conditions.
Introduction of the methosulfate group: This is achieved by reacting the quinoacridinium core with methyl sulfate under controlled conditions
Industrial production methods for RHPS 4 methosulfate are not widely documented, but the laboratory synthesis methods provide a basis for scaling up the production.
Chemical Reactions Analysis
RHPS 4 methosulfate undergoes several types of chemical reactions:
Oxidation: The compound can be oxidized under specific conditions, leading to the formation of various oxidation products.
Reduction: Reduction reactions can be performed using reducing agents, resulting in the formation of reduced derivatives.
Substitution: The compound can undergo substitution reactions, particularly at the fluorine atoms, leading to the formation of substituted derivatives
Common reagents and conditions used in these reactions include oxidizing agents like potassium permanganate, reducing agents like sodium borohydride, and nucleophiles for substitution reactions. The major products formed from these reactions depend on the specific conditions and reagents used.
Scientific Research Applications
RHPS 4 methosulfate has several scientific research applications:
Cancer Research: It is used to study the effects of telomerase inhibition on cancer cell proliferation and apoptosis. .
Telomere Biology: The compound is used to investigate the role of telomeres in cellular aging and cancer. .
Drug Development: RHPS 4 methosulfate is a lead compound for developing new anticancer drugs targeting telomerase and telomere structures
Mechanism of Action
RHPS 4 methosulfate exerts its effects by targeting the telomeric DNA G-quadruplex structures. By stabilizing these structures, it inhibits the activity of telomerase, an enzyme responsible for maintaining telomere length. This leads to telomere dysfunction, resulting in cell cycle arrest and apoptosis in cancer cells . The molecular targets involved include telomeric DNA and associated proteins.
Comparison with Similar Compounds
Structural and Functional Comparisons
Table 1: Key Properties of RHPS4 and Comparable G4 Ligands
Mechanistic Differences
- This compound vs. TRZ : this compound preferentially stabilizes antiparallel G4 structures, while TRZ targets parallel folds. Both inhibit telomerase at similar levels, but TRZ’s structural selectivity may benefit cancers with parallel G4 dominance .
- This compound vs. TMPyP4 : TMPyP4, a cationic porphyrin, requires higher concentrations (IC50 ~50 µM) for telomerase inhibition and exhibits lower cytotoxicity, making it safer but less potent .
- This compound vs.
- This compound vs. AIC : AIC selectively targets c-Myc promoter G4 structures, offering a distinct mechanism for c-Myc-driven cancers, whereas this compound broadly disrupts telomeric G4 .
Preclinical and Clinical Efficacy
- This compound: Shows potent anti-tumor activity in glioblastoma, melanoma, and breast cancer models. However, its cardiotoxicity and normal cell toxicity (IC50 = 5–15 µM in endothelial cells) limit clinical translation .
- TRZ: Limited data on in vivo efficacy, though its parallel G4 selectivity suggests niche applications .
- TMPyP4: Non-toxic at subcytotoxic doses but requires combination therapies for significant effects .
- Ligand #190 : Demonstrates superior toxicological profiles in preclinical studies, positioning it as a promising successor to this compound .
Combination Therapy Potential
- This compound synergizes with photon/carbon-ion radiation and Taxol, enhancing chromosomal damage and telomere fusion in glioblastoma . In contrast, TRZ and TMPyP4 lack robust evidence for such synergies.
- This compound’s radiosensitization fails in cancer stem-like cells (GSCs), underscoring context-dependent limitations .
Mitochondrial and Off-Target Effects
- This compound induces reversible mtDNA depletion and inhibits mitochondrial polymerase activity, complicating its safety profile .
Biological Activity
RHPS4, a pentacyclic acridinium compound, has garnered significant attention in cancer research due to its unique ability to interact with G-quadruplex (G4) DNA structures, particularly at telomeric regions. This interaction leads to telomere dysfunction and apoptosis in cancer cells, making this compound a promising candidate for anticancer therapy. This article delves into the biological activity of this compound, summarizing key findings from various studies, including data tables and case studies.
This compound primarily acts by stabilizing G-quadruplex structures within telomeric DNA, which inhibits telomerase activity and induces telomere uncapping. This results in DNA damage responses that are particularly pronounced in malignant cells but not in normal cells. The compound's mechanism can be summarized as follows:
- G-Quadruplex Stabilization : this compound binds to G4 structures, leading to the disruption of telomere architecture.
- Telomere Dysfunction : Induces telomere uncapping and subsequent DNA damage signaling.
- Apoptosis Induction : Triggers cell death pathways in cancer cells while sparing normal cells.
In Vitro Studies
- Cell Proliferation Inhibition : Studies have shown that this compound effectively inhibits cell proliferation in various cancer cell lines, including melanoma and colorectal cancer. A dose-dependent response was observed, with significant effects noted at concentrations as low as 0.1 µM .
- Cell Cycle Arrest : Flow cytometric analysis demonstrated that this compound induces a dose-dependent accumulation of cells in the S-G(2)/M phases of the cell cycle, leading to apoptosis .
- Telomere Damage Response : The treatment with this compound resulted in increased levels of γ-H2AX, a marker of DNA damage, indicating that telomere dysfunction occurs rapidly following treatment .
In Vivo Studies
- Tumor Growth Inhibition : In xenograft models, this compound demonstrated potent antitumor activity. For instance, in human melanoma xenografts, this compound treatment led to a reduction in tumor growth by approximately 40% compared to controls .
- Combination Therapy : When combined with chemotherapeutics like camptothecin, this compound enhanced the overall therapeutic effect, suggesting potential for combination therapies in clinical settings .
- Resistance Mechanisms : Tumors overexpressing protective telomeric proteins such as TRF2 and POT1 showed resistance to this compound treatment, highlighting the importance of these proteins in mediating the drug's efficacy .
Data Tables
Study | Cell Line | Concentration (µM) | Effect on Proliferation (%) | Apoptosis Index (%) |
---|---|---|---|---|
Melanoma | 0.1 | 60 | 9.2 | |
Colorectal | 0.5 | 75 | 12 | |
Melanoma | 1 | 50 | 15 |
Case Study 1: Melanoma Treatment
A study involving two melanoma cell lines derived from the same patient demonstrated that this compound was effective against both primary and metastatic tumors. While the primary line was sensitive to conventional chemotherapy (cisplatin), the metastatic line showed resistance; however, this compound reduced tumor weight by approximately 40% in this resistant line .
Case Study 2: Colorectal Cancer
In another study focusing on colorectal cancer xenografts, this compound was shown to significantly enhance sensitivity to camptothecin-based therapies. Mice treated with a sequence of irinotecan followed by this compound exhibited delayed tumor growth and improved survival rates compared to those receiving irinotecan alone .
Q & A
Q. What is the primary biochemical mechanism through which RHPS4 exerts its anti-cancer effects?
Answer: this compound selectively binds to G-quadruplex (G4) DNA structures, particularly in telomeres and mitochondrial DNA (mtDNA), stabilizing these regions and inhibiting telomerase activity. This disrupts DNA replication, induces replication fork stalling, and triggers DNA damage responses (DDR), leading to S-G2 phase cell cycle arrest and apoptosis. Key molecular markers include increased γH2AX foci (DNA damage) and activation of ATR/Chk2 pathways .
Q. How can researchers reconcile contradictory findings regarding this compound's dependence on mitochondrial DNA (mtDNA) for radiosensitization effects?
Answer: Contradictions arise from cell type-specific responses. To address this:
- Use mtDNA-depleted models (e.g., via EtBr treatment) to isolate nuclear vs. mitochondrial effects.
- Quantify mtDNA copy number via qPCR (targeting regions like CYTB/ND4) and assess clonogenic survival under varying this compound concentrations (0.1–2 µM).
- Studies show this compound retains radiosensitization in mtDNA-depleted cells, cell models (e.g., via EtBr treatment) and compare outcomes. Studies show this compound's radiosensitization persists without mtDNA, suggesting additional nuclear targets. Including qPCR for mtDNA regions and clonogenic assays under varying this compound concentrations can clarify context-dependent effects.
Q. What are the recommended experimental concentrations and treatment durations for this compound in in vitro studies?
Answer:
- Concentration range: 0.1–2 µM (IC50 = 0.33 µM for telomerase inhibition).
- Treatment duration: 24–72 hours for proliferation assays; 48–96 hours post-irradiation (2–6 Gy) for radiosensitization studies.
- Critical controls: Include solvent controls (e.g., DMSO) and validate via clonogenic assays or flow cytometry (BrdU/PI staining) .
Q. What methodological considerations are critical when assessing this compound-induced replication stress in telomeric vs. mitochondrial DNA?
Answer:
- Telomeric focus: Use chromatin immunoprecipitation (ChIP) for TRF1/2 proteins and BrdU labeling to map replication fork stalling.
- Mitochondrial focus: Employ mitochondrial-specific dyes (Mitotracker Deep Red) and quantify mtDNA via qPCR.
- Differentiation: Subcellular fractionation or CRISPR-based mtDNA depletion to isolate nuclear vs. mitochondrial effects .
Q. How does this compound influence cell cycle progression, and what molecular markers indicate its activity?
Answer: this compound induces S-G2 phase arrest by activating the ATR-Chk2-p21 axis. Key markers:
- Flow cytometry: BrdU/PI staining for cell cycle distribution.
- Western blot: Phosphorylated ATR (Ser428), Chk2 (Thr68), and γH2AX.
- Functional readouts: Reduced clonogenic survival and increased apoptosis (Annexin V/PI assays) .
Q. What strategies mitigate off-target effects when studying this compound's telomere-specific actions?
Answer:
- Competition assays: Co-treat with excess G4 oligonucleotides to confirm target specificity.
- Genetic controls: Use TRF2-knockdown cells to isolate telomere-specific effects.
- Negative controls: Non-telomeric G4 ligands (e.g., TMPyP4) to rule out general G4 stabilization .
Q. What are the key parameters for validating this compound's efficacy in preclinical models?
Answer:
- In vivo metrics: Tumor growth delay in xenografts post-RHPS4/radiation combo.
- Mitochondrial biomarkers: mtDNA copy number (qPCR) and citrate synthase (CS) activity in tissues.
- Toxicity monitoring: Liver/kidney function tests and hematological profiling .
Q. How should researchers address variability in this compound's anti-proliferative effects across cancer cell lines?
Answer:
- Dose-response curves: Test 0.1–10 µM this compound with clonogenic assays.
- Stratification: Group cells by telomerase activity (TRAP assay) and mtDNA content.
- Transcriptomics: RNA-seq to identify resistance pathways (e.g., ATR upregulation) .
Q. What controls are essential when evaluating this compound's impact on mitochondrial membrane potential?
Answer:
- Positive control: CCCP (carbonyl cyanide m-chlorophenyl hydrazone) to induce depolarization.
- Staining: JC-1 or TMRM dyes with flow cytometry.
- Functional validation: ATP production assays (luciferase-based) .
Q. What computational tools aid in predicting this compound's binding affinity to non-telomeric G4 structures?
Answer:
Properties
IUPAC Name |
4,16-difluoro-8,11,20-trimethyl-8-aza-20-azoniapentacyclo[11.7.1.02,7.09,21.014,19]henicosa-1(20),2(7),3,5,9,11,13(21),14(19),15,17-decaene;methyl sulfate | |
---|---|---|
Source | PubChem | |
URL | https://pubchem.ncbi.nlm.nih.gov | |
Description | Data deposited in or computed by PubChem | |
InChI |
InChI=1S/C22H17F2N2.CH4O4S/c1-12-8-16-15-10-13(23)4-6-18(15)26(3)22-17-11-14(24)5-7-19(17)25(2)20(9-12)21(16)22;1-5-6(2,3)4/h4-11H,1-3H3;1H3,(H,2,3,4)/q+1;/p-1 | |
Source | PubChem | |
URL | https://pubchem.ncbi.nlm.nih.gov | |
Description | Data deposited in or computed by PubChem | |
InChI Key |
VRWGYMXWYZBBGF-UHFFFAOYSA-M | |
Source | PubChem | |
URL | https://pubchem.ncbi.nlm.nih.gov | |
Description | Data deposited in or computed by PubChem | |
Canonical SMILES |
CC1=CC2=C3C(=C1)N(C4=C(C3=[N+](C5=C2C=C(C=C5)F)C)C=C(C=C4)F)C.COS(=O)(=O)[O-] | |
Source | PubChem | |
URL | https://pubchem.ncbi.nlm.nih.gov | |
Description | Data deposited in or computed by PubChem | |
Molecular Formula |
C23H20F2N2O4S | |
Source | PubChem | |
URL | https://pubchem.ncbi.nlm.nih.gov | |
Description | Data deposited in or computed by PubChem | |
Molecular Weight |
458.5 g/mol | |
Source | PubChem | |
URL | https://pubchem.ncbi.nlm.nih.gov | |
Description | Data deposited in or computed by PubChem | |
Retrosynthesis Analysis
AI-Powered Synthesis Planning: Our tool employs the Template_relevance Pistachio, Template_relevance Bkms_metabolic, Template_relevance Pistachio_ringbreaker, Template_relevance Reaxys, Template_relevance Reaxys_biocatalysis model, leveraging a vast database of chemical reactions to predict feasible synthetic routes.
One-Step Synthesis Focus: Specifically designed for one-step synthesis, it provides concise and direct routes for your target compounds, streamlining the synthesis process.
Accurate Predictions: Utilizing the extensive PISTACHIO, BKMS_METABOLIC, PISTACHIO_RINGBREAKER, REAXYS, REAXYS_BIOCATALYSIS database, our tool offers high-accuracy predictions, reflecting the latest in chemical research and data.
Strategy Settings
Precursor scoring | Relevance Heuristic |
---|---|
Min. plausibility | 0.01 |
Model | Template_relevance |
Template Set | Pistachio/Bkms_metabolic/Pistachio_ringbreaker/Reaxys/Reaxys_biocatalysis |
Top-N result to add to graph | 6 |
Feasible Synthetic Routes
Disclaimer and Information on In-Vitro Research Products
Please be aware that all articles and product information presented on BenchChem are intended solely for informational purposes. The products available for purchase on BenchChem are specifically designed for in-vitro studies, which are conducted outside of living organisms. In-vitro studies, derived from the Latin term "in glass," involve experiments performed in controlled laboratory settings using cells or tissues. It is important to note that these products are not categorized as medicines or drugs, and they have not received approval from the FDA for the prevention, treatment, or cure of any medical condition, ailment, or disease. We must emphasize that any form of bodily introduction of these products into humans or animals is strictly prohibited by law. It is essential to adhere to these guidelines to ensure compliance with legal and ethical standards in research and experimentation.