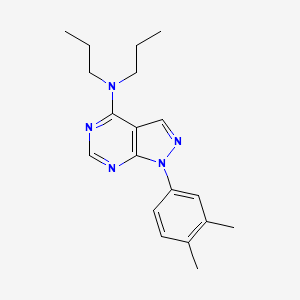
S3QEL-2
Overview
Description
S3QEL 2 is a chemical compound known for its ability to selectively inhibit the production of superoxide from the outer Q-binding site of mitochondrial complex III (site IIIQo). This compound does not affect oxidative phosphorylation or normal electron flux, making it a valuable tool in studying mitochondrial function and oxidative stress .
Mechanism of Action
Target of Action
S3QEL-2, also known as “1-(3,4-dimethylphenyl)-N,N-dipropyl-1H-pyrazolo[3,4-d]pyrimidin-4-amine”, is a suppressor of superoxide production from mitochondrial complex III . It potently and selectively suppresses site IIIQo superoxide production . Site IIIQo is the primary source of mitochondrial reactive oxygen species (ROS) and has been implicated in a broad range of ROS-mediated signaling and pathology .
Mode of Action
This compound acts by selectively inhibiting superoxide production at the IIIQo site of mitochondrial complex III . , ensuring that the energy metabolism of the cell remains unaffected.
Biochemical Pathways
The primary biochemical pathway affected by this compound is the mitochondrial electron transport chain, specifically at the IIIQo site of complex III . By suppressing superoxide production at this site, this compound modulates the production of ROS, which are both cellular signals and damaging oxidants . The suppression of ROS production can influence various cellular signaling pathways, including those related to hypoxic and oxidative stress .
Pharmacokinetics
It is known that this compound is a cell-permeable compound , suggesting that it can be absorbed and distributed within the body’s cells
Result of Action
This compound has been shown to inhibit HIF-1α accumulation , a transcription factor that plays a critical role in cellular responses to hypoxia. Additionally, this compound protects against ROS-induced, JNK-mediated cell stress in pancreatic β-cells and strongly mitigates the oxidative stress-induced apoptosis that limits the yield of functional β-cells from intact islets .
Action Environment
The effectiveness of this compound can be influenced by the metabolic context of the cell . For instance, the contribution of site IIIQo to overall ROS production can vary, and each S3QEL suppressed more strongly when the predicted contribution from site IIIQo was higher . This suggests that the cellular environment and metabolic state can influence the action and efficacy of this compound.
Biochemical Analysis
Biochemical Properties
S3QEL-2 interacts with the mitochondrial respiratory complex site III (IIIQo), where it selectively suppresses superoxide production . This interaction does not affect oxidative phosphorylation and normal electron flux .
Cellular Effects
This compound has been found to have significant effects on cellular processes. It inhibits HIF-1α accumulation , which is a key regulator of oxygen homeostasis in cells. Additionally, it protects against ROS-induced, JNK-mediated cell stress in pancreatic beta-cells .
Molecular Mechanism
The molecular mechanism of this compound involves its binding to the outer Q-binding site of mitochondrial respiratory complex site III (IIIQo). This binding suppresses the production of superoxide, a type of reactive oxygen species (ROS), without altering the normal electron flux or oxidative phosphorylation .
Temporal Effects in Laboratory Settings
It has been observed that this compound decreases the oxidative stress-induced apoptosis that limits the yield of functional β-cells from intact islets .
Metabolic Pathways
Given its role in suppressing superoxide production, it is likely to interact with pathways related to oxidative stress and mitochondrial function .
Subcellular Localization
Given its interaction with the mitochondrial respiratory complex, it is likely that it localizes to the mitochondria
Preparation Methods
The synthesis of S3QEL 2 involves several steps, including the preparation of intermediate compounds and the final coupling reaction. The detailed synthetic route and reaction conditions are typically proprietary and may vary depending on the manufacturer. Industrial production methods often involve optimizing these synthetic routes to ensure high yield and purity .
Chemical Reactions Analysis
S3QEL 2 primarily undergoes reactions related to its role as an inhibitor of superoxide production. It does not participate in typical oxidation, reduction, or substitution reactions commonly seen with other compounds. The major product formed from its reactions is the inhibition of superoxide production at site IIIQo, which is crucial for studying mitochondrial function and oxidative stress .
Scientific Research Applications
S3QEL 2 has a wide range of scientific research applications, including:
Chemistry: Used to study the mechanisms of superoxide production and its inhibition in mitochondrial complex III.
Biology: Helps in understanding the role of superoxide in cellular signaling and oxidative stress.
Medicine: Investigated for its potential therapeutic applications in diseases related to oxidative stress, such as neurodegenerative diseases and diabetes.
Industry: Utilized in the development of new drugs and therapeutic agents targeting mitochondrial dysfunction
Comparison with Similar Compounds
S3QEL 2 is unique in its selective inhibition of superoxide production at site IIIQo without affecting oxidative phosphorylation. Similar compounds include:
Myxothiazol: Inhibits site IIIQo but also affects oxidative phosphorylation.
Antimycin A: Induces superoxide production from site IIIQo and affects oxidative phosphorylation.
EUK-134: A mimetic of superoxide dismutase and catalase, used for comparison in studies involving oxidative stress
S3QEL 2 stands out due to its specificity and minimal impact on normal mitochondrial function, making it a valuable tool for research.
Biological Activity
S3QEL-2 (1-(3,4-Dimethylphenyl)-N,N-dipropyl-1H-pyrazolo[3,4-d]pyrimidin-4-amine) is a compound recognized for its ability to selectively suppress superoxide production from mitochondrial complex III, specifically at the Qo site. This article delves into the biological activity of this compound, highlighting its mechanisms, effects on various cell types, and implications for therapeutic applications.
This compound operates primarily by inhibiting superoxide production at the Qo site of mitochondrial complex III without disrupting normal electron transport or oxidative phosphorylation. This selectivity allows this compound to maintain mitochondrial function while reducing reactive oxygen species (ROS) generation.
- IC50 Value : The half-maximal inhibitory concentration (IC50) of this compound for superoxide production is approximately 1.7 μM .
1. Impact on ROS Production
This compound has been shown to significantly reduce hydrogen peroxide (H2O2) production in various cellular contexts:
- In human embryonic kidney (HEK-293) cells, this compound inhibited the accumulation of HIF-1α during hypoxic conditions, indicating a role in modulating cellular responses to oxidative stress .
2. Protection Against Oxidative Stress
This compound demonstrates protective effects against oxidative stress-induced apoptosis:
- In pancreatic β-cells exposed to tunicamycin, this compound mitigated caspase 3/7 activation and reduced cellular ROS levels, enhancing cell survival and function .
3. Effects on Hypoxia-Induced Signaling
This compound has been shown to modulate hypoxia-induced signaling pathways:
- It suppressed HIF-1α stabilization without affecting overall cellular oxidative phosphorylation, suggesting a unique mechanism that separates ROS signaling from metabolic disruption .
Table 1: Summary of Biological Activities of this compound
Implications for Therapeutic Applications
The ability of this compound to selectively inhibit superoxide production while preserving mitochondrial function makes it a promising candidate for therapeutic interventions in diseases characterized by oxidative stress and mitochondrial dysfunction. Its application could extend to conditions such as diabetes, neurodegenerative diseases, and ischemia-reperfusion injuries.
Properties
IUPAC Name |
1-(3,4-dimethylphenyl)-N,N-dipropylpyrazolo[3,4-d]pyrimidin-4-amine | |
---|---|---|
Source | PubChem | |
URL | https://pubchem.ncbi.nlm.nih.gov | |
Description | Data deposited in or computed by PubChem | |
InChI |
InChI=1S/C19H25N5/c1-5-9-23(10-6-2)18-17-12-22-24(19(17)21-13-20-18)16-8-7-14(3)15(4)11-16/h7-8,11-13H,5-6,9-10H2,1-4H3 | |
Source | PubChem | |
URL | https://pubchem.ncbi.nlm.nih.gov | |
Description | Data deposited in or computed by PubChem | |
InChI Key |
MGIAJZSCNPODRR-UHFFFAOYSA-N | |
Source | PubChem | |
URL | https://pubchem.ncbi.nlm.nih.gov | |
Description | Data deposited in or computed by PubChem | |
Canonical SMILES |
CCCN(CCC)C1=NC=NC2=C1C=NN2C3=CC(=C(C=C3)C)C | |
Source | PubChem | |
URL | https://pubchem.ncbi.nlm.nih.gov | |
Description | Data deposited in or computed by PubChem | |
Molecular Formula |
C19H25N5 | |
Source | PubChem | |
URL | https://pubchem.ncbi.nlm.nih.gov | |
Description | Data deposited in or computed by PubChem | |
Molecular Weight |
323.4 g/mol | |
Source | PubChem | |
URL | https://pubchem.ncbi.nlm.nih.gov | |
Description | Data deposited in or computed by PubChem | |
Retrosynthesis Analysis
AI-Powered Synthesis Planning: Our tool employs the Template_relevance Pistachio, Template_relevance Bkms_metabolic, Template_relevance Pistachio_ringbreaker, Template_relevance Reaxys, Template_relevance Reaxys_biocatalysis model, leveraging a vast database of chemical reactions to predict feasible synthetic routes.
One-Step Synthesis Focus: Specifically designed for one-step synthesis, it provides concise and direct routes for your target compounds, streamlining the synthesis process.
Accurate Predictions: Utilizing the extensive PISTACHIO, BKMS_METABOLIC, PISTACHIO_RINGBREAKER, REAXYS, REAXYS_BIOCATALYSIS database, our tool offers high-accuracy predictions, reflecting the latest in chemical research and data.
Strategy Settings
Precursor scoring | Relevance Heuristic |
---|---|
Min. plausibility | 0.01 |
Model | Template_relevance |
Template Set | Pistachio/Bkms_metabolic/Pistachio_ringbreaker/Reaxys/Reaxys_biocatalysis |
Top-N result to add to graph | 6 |
Feasible Synthetic Routes
Disclaimer and Information on In-Vitro Research Products
Please be aware that all articles and product information presented on BenchChem are intended solely for informational purposes. The products available for purchase on BenchChem are specifically designed for in-vitro studies, which are conducted outside of living organisms. In-vitro studies, derived from the Latin term "in glass," involve experiments performed in controlled laboratory settings using cells or tissues. It is important to note that these products are not categorized as medicines or drugs, and they have not received approval from the FDA for the prevention, treatment, or cure of any medical condition, ailment, or disease. We must emphasize that any form of bodily introduction of these products into humans or animals is strictly prohibited by law. It is essential to adhere to these guidelines to ensure compliance with legal and ethical standards in research and experimentation.